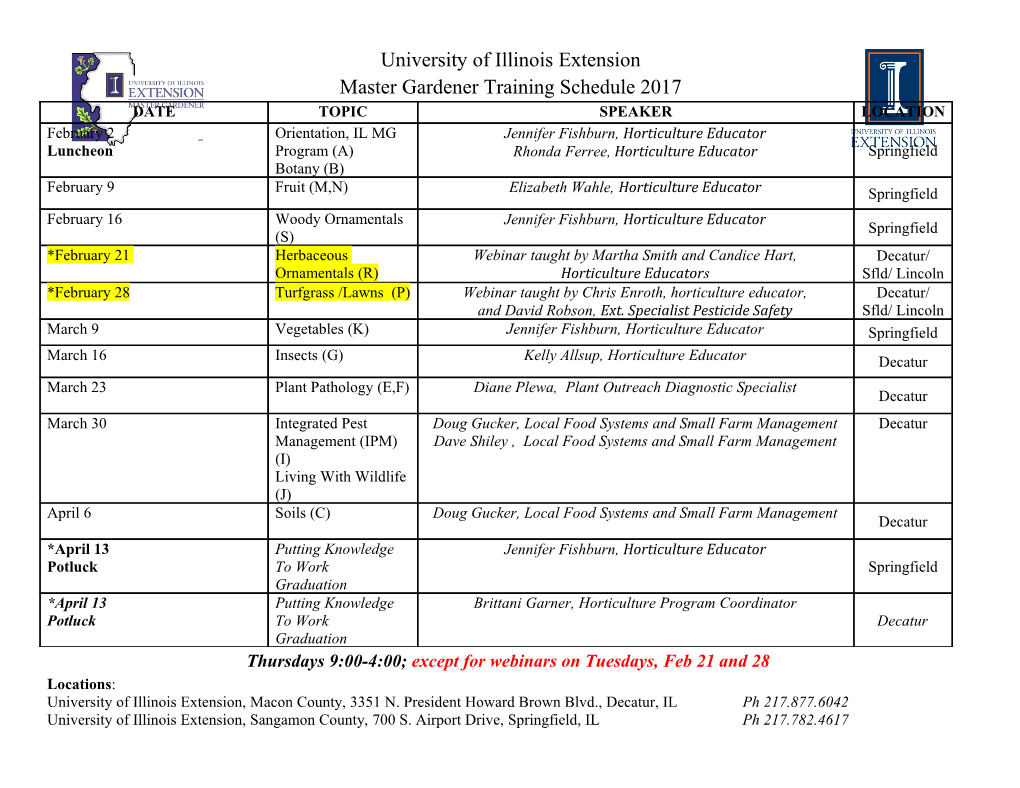
Journal of Power Sources 277 (2015) 169e179 Contents lists available at ScienceDirect Journal of Power Sources journal homepage: www.elsevier.com/locate/jpowsour Component-/structure-dependent elasticity of solid electrolyte interphase layer in Li-ion batteries: Experimental and computational studies * Hosop Shin a, Jonghyun Park a, , Sangwoo Han a, Ann Marie Sastry b, Wei Lu a a Department of Mechanical Engineering, University of Michigan, Ann Arbor, MI 48109, USA b Sakti3, Inc., Ann Arbor, MI 48108, USA highlights Characterization of inhomogeneity in SEI elasticity via an AFM/XPS analysis. Calculation of elastic moduli of key SEI layer components. Validation of experimental observations through atomistic calculations. article info abstract Article history: The mechanical instability of the Solid Electrolyte Interphase (SEI) layer in lithium ion (Li-ion) batteries Received 18 October 2014 causes significant side reactions resulting in Li-ion consumption and cell impedance rise by forming Received in revised form further SEI layers, which eventually leads to battery capacity fade and power fade. In this paper, the 22 November 2014 composition-/structure-dependent elasticity of the SEI layer is investigated via Atomic Force Microscopy Accepted 26 November 2014 (AFM) measurements coupled with X-ray Photoelectron Spectroscopy (XPS) analysis, and atomistic Available online 26 November 2014 calculations. It is observed that the inner layer is stiffer than the outer layer. The measured Young's moduli are mostly in the range of 0.2e4.5 GPa, while some values above 80 GPa are also observed. This Keywords: SEI wide variation of the observed elastic modulus is elucidated by atomistic calculations with a focus on Elasticity chemical and structural analysis. The numerical analysis shows the Young's moduli range from 2.4 GPa to Molecular dynamics 58.1 GPa in the order of the polymeric, organic, and amorphous inorganic components. The crystalline AFM inorganic component (LiF) shows the highest value (135.3 GPa) among the SEI species. This quantitative observation on the elasticity of individual components of the SEI layer must be essential to analyzing the mechanical behavior of the SEI layer and to optimizing and controlling it. © 2014 Elsevier B.V. All rights reserved. 1. Introduction during subsequent cycles. It enables a Li-ion battery to maintain its good capacity retention even if the battery operates below the The performance of a Li-ion batterydsuch as irreversible ca- reduction potential of electrolytes. This is the case, however, only if pacity loss, 1st cycle columbic efficiency, and cycle/calendar lifedis a perfect and stable SEI layer is formed and maintained during the closely related to the properties of the solid electrolyte interphase cycling. In reality, the SEI layer does not keep its initial configura- (SEI) layer [1e5]. The SEI layer that formed on the negative elec- tion and properties [6]. The aging or degradation process of the SEI trode during the initial few cycles is known as an ionic conductor layer proceeds to a low extent and at a low rate for the whole cycle/ for lithium but not an electronic conductor, thereby it protects the calendar life of Li-ion batteries [5,6]. For instance, dissolution/ electrode from further reductive decomposition of the electrolyte breakdown, conversion, and growth of the SEI layer can occur during prolonged storage and cycling, especially at high tempera- tures [3,5,7e9]. The phenomena cause changes in SEI layer prop- * Corresponding author. Present address: Department of Mechanical and Aero- erties, such as composition and morphology, so that the electrons space Engineering, Missouri University of Science and Technology, Rolla, MO 65409, and solvents could be permeable in the defective SEI layer, leading USA. to additional electrolyte decomposition. Continuous E-mail address: [email protected] (J. Park). http://dx.doi.org/10.1016/j.jpowsour.2014.11.120 0378-7753/© 2014 Elsevier B.V. All rights reserved. 170 H. Shin et al. / Journal of Power Sources 277 (2015) 169e179 decomposition reactions promote further SEI formation or SEI SEI layer on a silicon anode was observed by adding the silane reconstruction, thickening the SEI layer and consuming cyclable additive in the electrolyte [19]. It was also found that the Young's lithium-ions. It has been shown that the SEI growth rate slows with modulus of the SEI layer on an MnO anode changes during the SEI time but never stops entirely during long-term storage and cycling formation process [20]. In addition to experimental observations, [5]. However, if the initially formed SEI layer has desirable and atomistic simulations were used to predict the elastic modulus of effective properties for SEI stability, the SEI growth rate can be the SEI layer. A molecular dynamics simulation using the ReaxFF diminished or even come close to stopping entirely, improving the potential suggested that ethylene carbonate (EC)-based SEI layer is capacity retention of Li-ion batteries. Thus, having an under- stiffer than the dimethyl carbonate (DMC)-based SEI layer [21]. standing and clarification of the SEI properties in terms of me- As mentioned earlier, the composition and structure of the SEI chanical and chemical aspects is the first important step in layer is quite dependent on the system including the anode mate- achieving the stability of the SEI layer, and eventually the rial, the electrolyte, and the initial formation environment. enhancement of the cycle/calendar life of Li-ion batteries. Furthermore, the mechanical properties of the SEI layer are closely Many efforts have been made recently to elucidate the structure, related to the composition and structure of the SEI layer. Previous morphology, chemical composition, and thickness of the SEI layer studies, however, have not provided SEI elasticity based on its on a negative electrode. Inhomogeneous mosaic-type or chemical composition and structure [19,20]. Consequently, a study multilayered-type SEI structure models have been proposed based on the component-/structure-dependent SEI elasticity can provide on the SEI layer formation process via 2D surface reduction [10,11]. useful guidance for building a more robust SEI layer. A 3D SEI model, including the intercalation of decomposition Previous experimental studies have used the probe-based products into the graphite interlayer, as well as the attachment of indentation technique to investigate elastic properties of the SEI the products to the graphite surface, has been also suggested [12]. layer [19,20]. Nanoindenter and AFM have been widely used for the Although no consensus on the detailed SEI structure has yet been probe-based nano-indentation technique to characterize the nano- reached, it is generally accepted that the SEI layer is composed of mechanical properties of various materials. However, the technique two distinct layers: a thin, dense layer of inorganic species close to is comparatively very slow, which makes detailed topographical the electrode side, and a thick, porous layer of organic and poly- mapping difficult. In addition, relatively large deformation depths meric compounds close to the electrolyte side. The inorganic spe- can lead a sample getting damaged, resulting in a poor lateral cies such as lithium fluoride (LiF), lithium carbonate (Li2CO3), and resolution. The large deformation depth can lead the measured lithium oxide (Li2O) are typically believed to exist as a poly- values to be unreliable due to the substrate effect, since SEI layer crystalline or crystalline phase, while the organic components such thickness is typically very thin. It is commonly believed that the as dilithium ethylene dicarbonate ([CH2OCO2Li]2,Li2EDC), lithium indentation depth should be less than a tenth of the film thickness methyl carbonate (LiOCO2CH3, LiMC), and lithium ethyl carbonate to avoid the substrate effect [22]. The PeakForce Quantitative (LiOCO2C2H5, LiEC) are commonly reported to present as a form of Nanomechanical (PF-QNM) mode with AFM, which was recently amorphous structure [9,13,14]. In addition, the formation of a long- introduced, has allowed quantitative mechanical property mapping chain oligomer or polymeric compound such as polyethylene oxide at the nanoscale with a high resolution and fast scanning for a range (PEO) has also been reported [14,15]. The chemical composition of material firmness, from soft to hard [23e29]. It directly controls the the SEI layer is dependent on the solvents and salts used as the maximum normal force to be constant, enabling small load forces electrolytes. The thickness of the SEI layer has been observed to be with very small deformation depths of a sample, which can mini- in the range of tens to hundreds of nanometers [13,16]. Since SEI mize the effect of a substrate on the measured modulus [22]. thickness as well as SEI composition can be influenced by artifacts In this work, SEI elasticity is investigated based on a coupled in measurements and different experimental conditions, the re- technique: AFM with PF-QNM mode and X-ray photoelectron ported values vary from one another. spectroscopy (XPS) measurements. Also, atomistic calculations are While numerous studies have been aimed at elucidating SEI conducted to decompose the component and structure effects on formation mechanisms and physical/chemical properties of the SEI the mechanical properties of the constituents of the SEI layer, layer, relatively little attention has been paid to the mechanical which is not possible in the measurement. properties of the SEI layer, especially SEI elasticity. During the repeated cycles, the particles of the negative electrode experience 2. Methods volume change, stress, and pressure build-up due to intercalation- induced strain and gas production, which can initiate the physical 2.1. Experimental procedure damage or the instability of the SEI layer as well as the particle surface of the electrode [16,17]. It has recently been observed that 2.1.1. Sample preparation stress change at the surface of a negative electrode is closely related A 2032 type-coin cell (MTI Corp.) was assembled in an argon- to the SEI layer formation [18].
Details
-
File Typepdf
-
Upload Time-
-
Content LanguagesEnglish
-
Upload UserAnonymous/Not logged-in
-
File Pages11 Page
-
File Size-