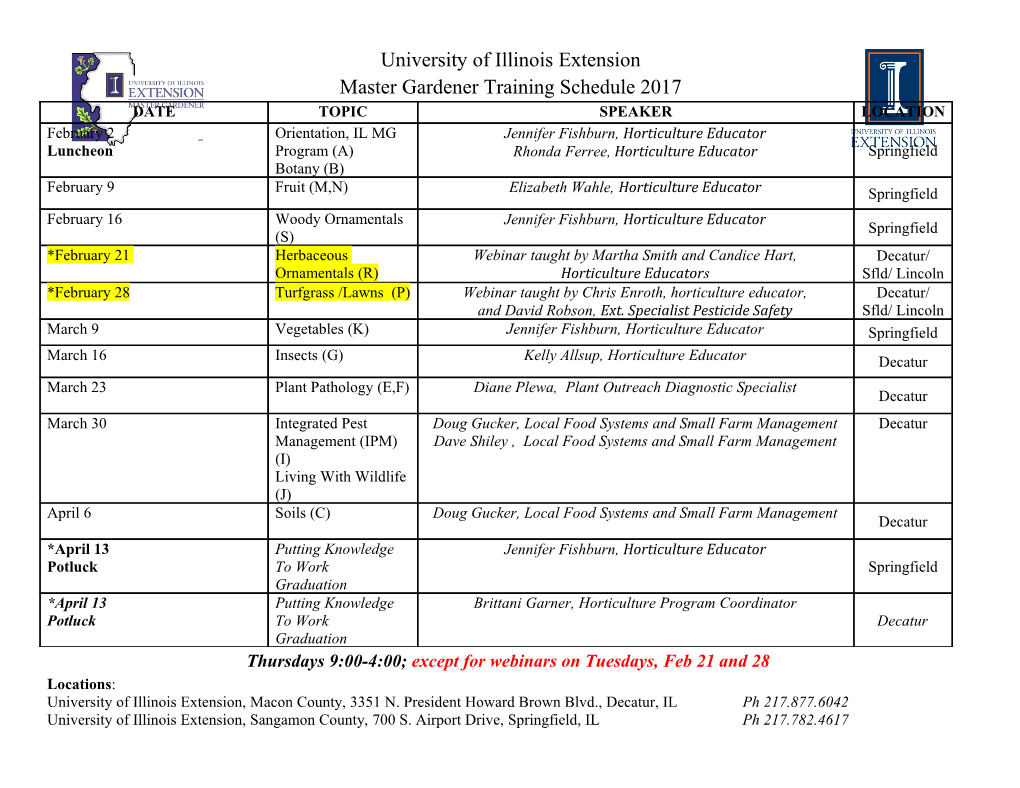
Biological Journal of the Linnean Society, 2012, 105, 607–622. With 8 figures Prey capture in lizards: differences in jaw–neck–forelimb coordination STÉPHANE J. MONTUELLE1*, ANTHONY HERREL2, PAUL-ANTOINE LIBOUREL3, SANDRA DAILLIE2 and VINCENT L. BELS2 1Ohio University, Heritage College of Osteopathic Medicine, Department of Biomedical Sciences, Athens, OH, USA 2Muséum National d’Histoire Naturelle, Département Ecologie et Gestion de la Biodiversité, UMR 7179, Paris, France 3Université Lyon 1, Institut fédératif des Neurosciences, UMR 5167, Lyon, France Received 26 August 2011; revised 25 September 2011; accepted for publication 25 September 2011bij_1809 607..622 Although prey capture is thought to be based on the coordinated movements of the jaw and locomotor apparatus (i.e. the vertebral column and the limbs), jaw–neck–forelimb coordination has never been compared among related species. The kinematics of jaw, neck, and forelimb movements were recorded in lizards that use jaw prehension: Gerrhosaurus major, Tupinambis merianae, Varanus niloticus, and Varanus ornatus. These species provide a comparative framework to discuss the influence of morphological differences and ecological convergence on the jaw–neck–forelimb coordination patterns. Jaw–neck–forelimb coordination was quantified by determining whether maximum neck elevation and maximum forelimb flexion are synchronized with either the start of jaw opening or maximum gape. Significant differences in the jaw–neck–forelimb coordination pattern among species were observed, with maximum neck elevation being synchronized with the start of jaw opening in varanids, whereas in T. merianae and G. major, it is achieved closer to maximum gape. Differences in locomotor–feeding integration are suggested to be related to dietary specializations, and as such may play a role in feeding adaptation. The jaw–neck–forelimb coordination pattern used by varanids may indeed be advantageous to prepare a quick strike triggered from further away, providing a critical advantage when feeding on evasive prey. © 2012 The Linnean Society of London, Biological Journal of the Linnean Society, 2012, 105, 607–622. ADDITIONAL KEYWORDS: feeding – integration – kinematics – Teiids – varanids. INTRODUCTION 2000b; Bels, 2003; Reilly & McBrayer 2007), sala- manders (for a review see Wake & Deban 2000), frogs, Living organisms exploit food resources to obtain and toads (for a review see Nishikawa 2000). The nutrients and the energy needed to function and performance of predators using tongue prehension is survive. Among the various components of feeding primarily based on the speed and accuracy of tongue behaviour, food acquisition is important as it includes projection jointly with the adhesive capacity of the stage during which the organism establishes the tongue (Wainwright, Kraklau & Bennett, 1991; contact with the food item. In animals feeding on Lappin et al., 2006; Deban et al., 2007). In other mobile prey (e.g. carnivores, insectivores), the ability tetrapods, the jaws are the elements through to decrease predator–prey distance is thus critical. which predator–prey contact is established (e.g. cro- Various predators among tetrapod vertebrates use the codylians, snakes, and autarchoglossan lizards; for a hyolingual apparatus to decrease predator–prey dis- review see Cleuren & de Vree 2000; Cundall & tance and catch prey, such as iguanian lizards (for a Greene, 2000; Schwenk, 2000b). During jaw prehen- review see Bels, Chardon & Kardong, 1994; Schwenk, sion, prey acquisition results from the movement of the head with opened jaws towards the prey, leading *Corresponding author. E-mail: [email protected] to the subsequent contact between the jaws of the © 2012 The Linnean Society of London, Biological Journal of the Linnean Society, 2012, 105, 607–622 607 608 S. J. MONTUELLE ET AL. predator and the prey. In snakes, for instance, the nation, optimal gape angle at contact might not be extension of the vertebral column, especially in achieved (e.g. jaws are not open wide enough when the cervical region, has been demonstrated to gener- predator–prey contact occurs), leading to an unsuc- ate the thrust necessary to cover predator–prey dis- cessful capture attempt. Previous studies of jaw pre- tance (Frazzetta, 1966; Janoo & Gasc, 1992; Kardong hension highlight the role of the cervical vertebrae in & Bels, 1998; Cundall & Deufel, 1999; Alfaro, 2003; snakes (Kardong & Bels, 1998; Cundall & Deufel, Young, 2010; Herrel et al., 2011). 1999; Alfaro, 2003; Young, 2010), but the coordination The functional analysis of feeding behaviour pro- of jaw movements with those of the locomotor elements vides meaningful insights into the evolution of the remains poorly quantified in other groups (but see form and function of anatomical structures (e.g. Bock Montuelle, Daghfous & Bels, 2008; Montuelle et al., & von Wahlert, 1965; Frazzetta, 1994; Schwenk, 2009a). Consequently, the present study aims to dem- 2000a). Indeed, the morphology of the feeding ele- onstrate the role of locomotor-feeding integration ments (i.e. the skull, the jaw, and the hyolingual during jaw prehension in lizards. apparatus) is shaped by the adaptive pressures of their Jaw prehension is a key feature of the evolution of respective function. For instance, the anatomy of the the squamate lineage. In the classical phylogene- hyolingual apparatus may evolve to enhance tongue tic hypothesis (based on morphological evidence; elongation (e.g. Schwenk, 1985; Delheusy, Toubeau & Fig. 1A), the evolution of jaw prehension is considered Bels, 1994; Sherbrooke & Schwenk, 2008; Herrel et al., to have ‘released’ the hyolingual apparatus from its 2009). Similarly, the jaw apparatus and the skull itself constraints during feeding, allowing for specialization are shaped with respect to diet to generate the towards other functions such as vomeronasal adequate bite force (e.g. Herrel, Aerts & de Vree, 1998; chemoreception (Wagner & Schwenk, 2000; Schwenk Herrel et al., 2001a, 2007; Herrel, O’Reilly & Rich- & Wagner, 2001; Vitt et al., 2003). In the recent phy- mond, 2002; Metzger & Herrel, 2005; Therrien, 2005; logenetic hypothesis (based on molecular evidence; Huber et al., 2009; Freeman & Lemen, 2010; Chris- Fig. 1B), jaw prehension is considered to be the ances- tiansen, 2011). Yet, the movements of the feeding tral prey capture mode (Lee, 2005; Vidal & Hedges, elements need to be coordinated with each other to 2005). Thus in both cases, the functional characteris- ensure the success of prey capture performance (e.g. tics of jaw prehension will probably contribute in our Herrel et al., 2001b; Schwenk, 2001; Bels, 2003; understanding of the evolution of feeding in the squa- Higham, 2007; Wainwright, Mehta & Higham, 2008). mate lineage. To date, locomotor-feeding integration The crucial aspect of feeding in an adaptive context has been demonstrated during jaw prehension in one thus concerns the movements of the feeding system as squamate lizard, Gerrhosaurus major Duméril 1851 an integrated whole, rather than of the feeding ele- (Scleroglossa, Autarchoglossa, Scincoidea; Montuelle ments independently. Consequently, changes in the et al., 2009a). Here, our objective is to compare jaw– form and function of one element may affect the other neck–forelimb integration in this species with other elements, and the adaptation of anatomical elements lizards that use jaw prehension: Tupinambis meri- is under the selective pressures that stem from the anae Duméril & Bibron 1839 (Scleroglossa, Autar- functionality of the entire system (Wagner & Schwenk, choglossa, Lacertoidea, Teiidae), Varanus niloticus 2000; Schwenk & Wagner, 2001). In the case of igua- Linnaeus 1758, and V. ornatus Daudin 1803 (Sclero- nian lizards that use tongue prehension, the integra- glossa, Autarchoglossa, Varanoidea, Varanidae). Tupi- tion of the jaws and the hyolingual apparatus is such nambis merianae, V. niloticus, and V. ornatus were that the feeding system is suggested to be an evolu- selected because they share comparable ecological tionarily stable configuration (Wagner & Schwenk, and behavioural features associated with feeding: 2000; Schwenk & Wagner, 2001). However, relatively an omnivorous diet (Presch, 1973; Losos & Greene, little is known about the functional basis of jaw 1988; Cooper, 2002), and similarities in foraging mode prehension in lizards. (Cooper, 1995; Thompson, 1995), prey detection From a functional perspective, prey prehension (Cooper, 1989, 1990, 1996; Yanosky, Iriart & Mercolli, relies on the positioning of the head and jaws according 1993; Kaufman, Burghardt & Phillips, 1996; Cooper to the prey characteristics. As such, jaw prehension is & Habegger, 2001; Chiszar et al., 2009), and transport achieved by the integration of the movements of the mode (Elias, McBrayer & Reilly, 2000; McBrayer & feeding elements (i.e. the jaws) with the locomotor Reilly, 2002b; Montuelle et al., 2009b; Schaerlaeken elements, especially the anterior locomotor elements et al., 2011). Morphological similarities in skull shape such as the forelimbs and the cervical vertebrae (Mon- and body proportions have also been documented tuelle et al., 2009a). Jaw movements must indeed be (Vitt & Pianka, 2004; Stayton, 2005). Given these synchronized with locomotor movements so that the similarities in morphological, behavioural, and eco- velocity of the head and the opening angle of the jaws logical traits, functional convergence in locomotor– result in predator–prey contact. Without such coordi- feeding integration
Details
-
File Typepdf
-
Upload Time-
-
Content LanguagesEnglish
-
Upload UserAnonymous/Not logged-in
-
File Pages16 Page
-
File Size-