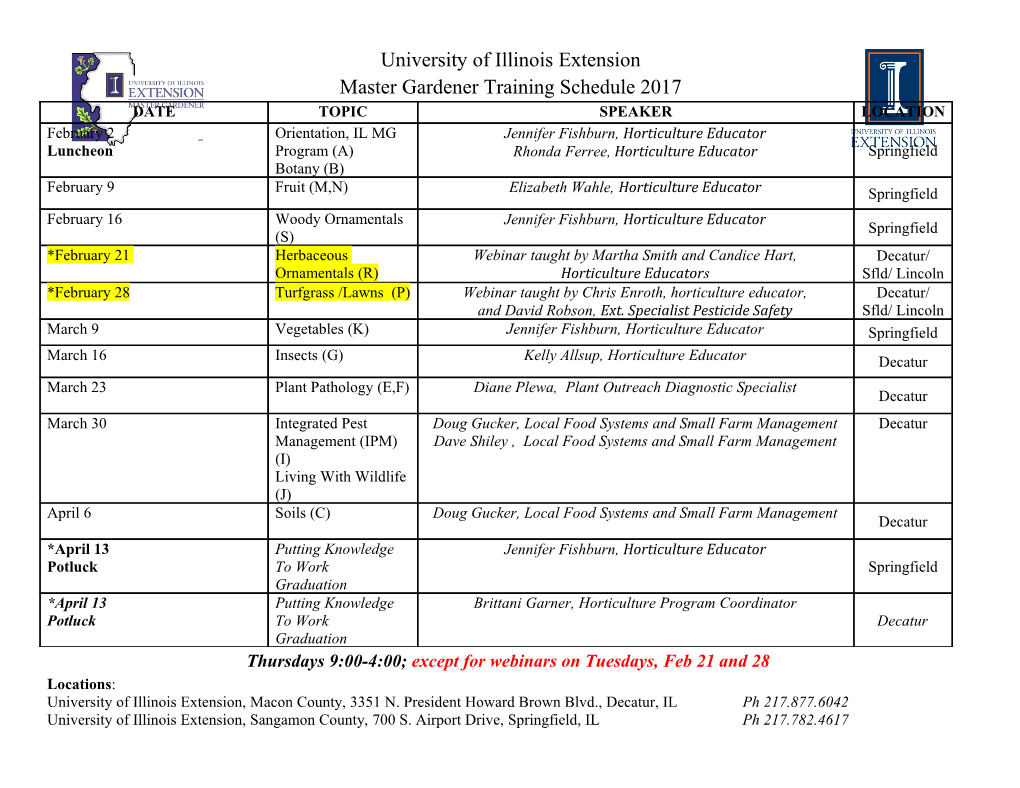
Small Spacecraft Overview Mr. Stevan M. Spremo, Mr. Alan R. Crocker, and Dr. Tina L. Panontin NASA Ames Research Center Summary Small spacecraft play a major role in earth, lunar, planetary, stellar, and interstellar discoveries. As technologies improve, instruments scale down in size, and their advantages in reduced cost and development time continue to attract investment, small satellites1 will play an even more important role. Today, the growth rate of small spacecraft utilization is limited by the availability of affordable launch opportunities. Introduction Small spacecraft have been an integral part of space study, exploration, and commercialization since humankind’s first steps into low Earth orbit with Sputnik-1 and Explorer-1. As defined in Table 1, Spacecraft Class Definition Wet Mass, kg Large Spacecraft ≥1000 Medium Spacecraft 500-1000 Mini Spacecraft 100-500 * Small Spacecraft Micro Spacecraft 10-100 Nano Spacecraft 1-10 }Cubesats Pico Spacecraft 0.01-1 Femto Spacecraft 0.01-0.01* * organization-dependent upper thresholds vary from 180 to 500 kg ** organization-dependent upper thresholds vary from 0.01 to 0.1kg Table 1 Spacecraft classification based on wet mass “small spacecraft” are considered to be those with wet masses below 500kg. Although the 500 to 1000 kg mass range is defined technically as a “medium” spacecraft class, it is common to omit reference to medium classifications and refer to a spacecraft as either “small” or “large”. 1 The term “satellite”, commonly used in describing an artificial object in orbit around the earth or other celestial body, can also refer to a spacecraft on an escape trajectory to eventually exit our solar system. Development of small satellite platform standards have created new opportunities in the nanosatellite market. The standard “one unit” (1U) cubesat spacecraft, illustrated in Figure 1, is a cube 10 cm on each side with a mass of approximately 1 kg. (CubeSat Program, 2014). Cubesats can be composed of a single cube (a “1U” cubesat) or several cubes combined forming, for instance, 3U, 6U or even 27U integrated units. Representative configurations and sizes are compared in Figure 2. Figure 1 Typical 1U Cubesat Most Cubesat masses qualify them as nanosatellites; however, cubesats less than 1 kg may be considered pico spacecraft. Practical femtosats have not yet entered the marketplace, but attempts to develop and launch such “ChipSats” systems are underway. (Jones, 2016) Figure 2 Comparative size of 1U, 3U, and 6U Cubesats Although their mass and volume constraints can significantly limit power, propulsion, and communication subsystem sizes, small spacecraft do provide certain advantages. With generally lower complexity and fewer payloads, small satellites can be produced at an increased cadence than larger ones. Rapid development provides organizations with more agility in meeting requirements and adapting to new technologies. For instance, the Department of Defense Operationally Responsive Space (ORS) program pursues small satellites to meet its primary goal to rapidly assemble, test, and launch satellites in support of warfighters. Rapid development can also drive costs down such that use of small satellites may allow more frequent research missions and technology demonstrations. Low cost developments can also provide opportunities for engineering and project management education and capability maturation. Cubesats, in particular, are often used as educational and technology demonstration platforms. History and Evolution of Small Spacecraft Small spacecraft are not a new phenomenon but rather are the original class of space vehicle and a cornerstone of the United States space program. The first artificial satellites – the Soviet Union’s Sputnik 1 and The United States’ Explorer 1 – were small satellites. More than 50 small spacecraft followed as part of NASA’s Explorer Program – missions investigating earth science, astronomy and heliophysics. (NASA, 2017). Though small in size, complexity, and cost, NASA's Explorer spacecraft were well-engineered and highly reliable. Many continued to operate for 5 or more years; the longest-lived, IMP-8, operated for 33 years. (NASA, 2017) Small spacecraft missions were not limited to just the Explorer Program. Many of the early interplanetary Mariner and Pioneer spacecraft may be categorized as small spacecraft. NASA’s Apollo Program deployed two small lunar satellites as part of the Apollo 15 and 16 missions of the early 1970’s. Organizations outside of NASA, including the Department of Defense, academia, and foreign governments, successfully developed and operated small spacecraft as well. NASA began the Small Explorer Program (SMEX), in 1989 as a follow-on to the Explorer Program. Cost capped at $120M (FY17 dollars), with a primary goal of utilizing small spacecraft to conduct mission in astrophysics, space physics and upper atmospheric science, the SMEX program was also to usher in a new generation of engineers through apprenticeship with experienced spacecraft developers. A decade later, NASA initiated the University-class explorer (“UNEX”) program to conduct missions at a per mission cost of less than $15M (FY17 dollars) that provides even more "hands-on" training ground for future spacecraft developers. Figure 3 shows the growing variety of NASA’s small spacecraft over many decades. Figure 3 Small spacecraft mass trends over the past six decades There are many examples of smallsats that provide cost-effective platforms for astrophysics, heliophysics, and Earth science research. One is the 200 kg Interface Region Imaging Spectrograph (IRIS) spacecraft that provides important insights into our Sun. Another is the 385 kg Stardust mission, launched in 1999, that collected comet dust samples and returned those samples to Earth. The Cyclone Global Navigation Satellite System (CYGNSS), launched in 2016, placed 8 microsatellites in low Earth orbit to study the formation and intensity of tropical cyclones and hurricanes. (NASA, 2016) The Time History of Events and Macroscale Interactions during Substorms (THEMIS) mission launched a constellation of 5 smallsats to study Earth’s magnetosphere. Military and commercial interest in smallsats has grown as well. In 2005, the 100 kg U.S. Air Force XSS-11 spacecraft demonstrated the ability to rendezvous with and repair another satellite. (David, 2005). In 1999, the cubesat standard revolutionized the secondary payload industry, replacing non-functional "ballast mass" with small, low cost spacecraft and creating a new, diverse community of spacecraft developers. Cubesats provide low-cost access to space, enabling research and educational projects that might not otherwise be possible using “traditional” methods. Their low cost make cubesat educational programs accessible from the elementary school to university levels. Cubesat design, launch, and operation programs are a growing part of STEM curricula in K-12 schools, community colleges, and universities. A cubesat dispenser design, the Poly-Picosat On-Orbit Deployer (P-POD) shown in Figure 4, emerged in parallel with the cubesat standard to enable easy integration with existing launch vehicles. The mechanically robust dispenser accommodated a pack of 3 1U spacecraft until commanded to eject the cubesats with a spring-actuated “foot”. Today, multiple cubesat dispenser options are available as open design standards and proprietary commercial solutions. Figure 4 P-POD Cubesat Launcher Beyond educational access to space, cubesats provide a low-cost, yet effective, means to demonstrate new technologies. For example, in 2012, NASA’s PhoneSat spacecraft demonstrated the application of COTS technologies for affordable spacecraft. NASA has also utilized cubesats for space biology experimentation since 2006. The U.S. Army’s Operational Nanosatellite Effect (ONE) program began investigating the use of cubesat platforms in 2011 (Brinton, 2011). Cubesats may also begin to play support roles in larger missions, for example, NASA’s InSight mission to Mars will use two Mars Cube One (MarCO) spacecraft to provide communications relay functions during InSight’s entry, descent and landing. (Matousek, 2014) Low cost Earth imaging is an emerging commercial market that benefits from small satellite capabilities. Several new companies plan to leverage the simplicity, modularity and affordability of cubesats to create satellite constellations. (Bandyopadhyay, Subramanian, Foust, Morgan, & Chu, 2015) One such constellation already includes over 100 operating 3U cubesats. From low Earth orbit, these satellites may provide Earth imaging at the maximum resolution allowed under U.S. export law. Government cubesat mission costs vary from $3 to $30 million and are typically determined by the scope of scientific capability, complexity, propulsion, control system type, and radiation tolerance requirements. A summary of selected NASA cubesat missions is provided in Table 2. Table 2 Example NASA Cubesat missions Figure 5 illustrates the quickly growing number and diversity of missions utilizing nano and micro satellites. Earth observation and science areas have grown significantly since 2013. Overall, the number of cubesat launches have seen large increases, as shown in Figure 6. The rate has remained high since 2013—a record 103 small satellites were launched simultaneously on an Indian Polar Satellite Launch Vehicle in Feb. 2017. The increased cubesat launch rate reflects the expanding viability of these platforms for research and commercial activities, aided in part
Details
-
File Typepdf
-
Upload Time-
-
Content LanguagesEnglish
-
Upload UserAnonymous/Not logged-in
-
File Pages21 Page
-
File Size-