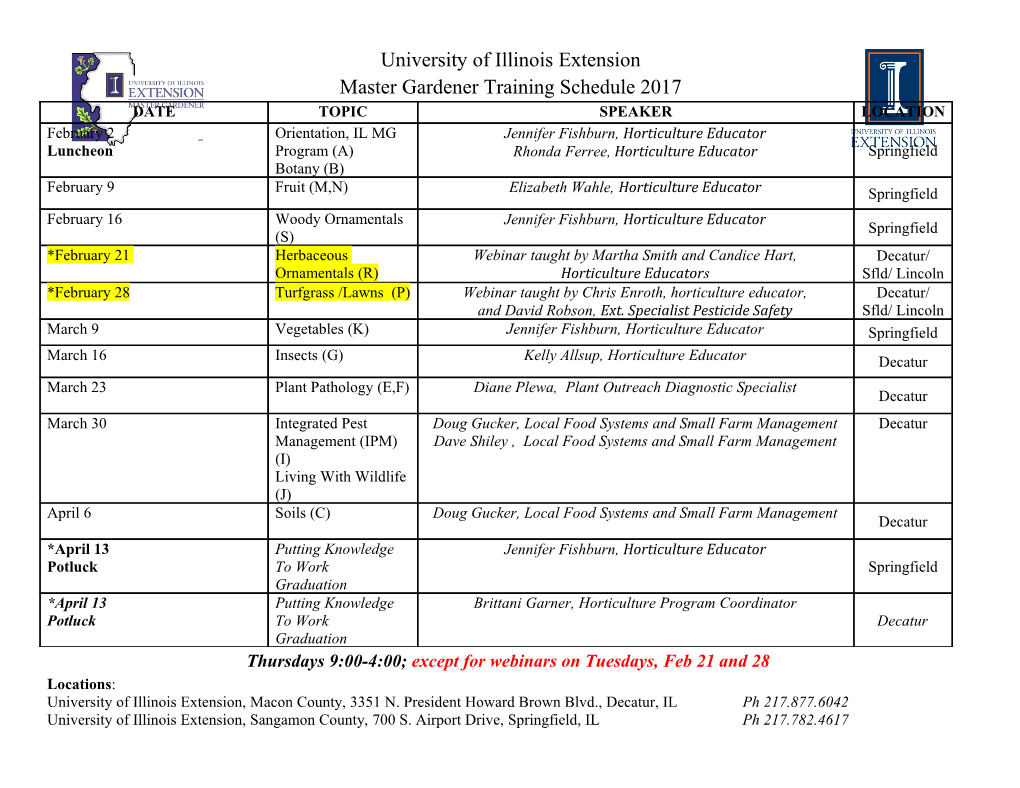
Biophysical Journal Volume 94 March 2008 2333–2342 2333 Differential Evanescence Nanometry: Live-Cell Fluorescence Measurements with 10-nm Axial Resolution on the Plasma Membrane Saveez Saffarian and Tomas Kirchhausen Department of Cell Biology and Immune Disease Institute, Harvard Medical School, Boston, Massachusetts ABSTRACT We present a method to resolve components within a diffraction-limited object by tracking simultaneously the average axial positions of two different sets of fluorescent molecules within it. The axial positions are then subtracted from each other to determine the separation of the two sets of fluorophores. This method follows the dynamic changes in the separation of the two sets of fluorophores with freely rotating dipoles using sequential acquisitions with total internal reflection and wide-field illumination, and it can be used to measure the formation of small structures on living cells. We have verified that we can achieve a resolution of 10 nm, and we have used the method to follow the location of clathrin and its adaptor AP-2 as they are recruited to a diffraction-limited coated pit during its assembly at the plasma membrane. We find a gradually increasing axial separation between the centroids of clathrin and AP-2 distribution, up to a final value of 30 nm just before coated-pit pinching and formation of the coated vesicle. INTRODUCTION The finite size of the diffraction-limited observation volume fluorophore can be recorded (23,24). This principle is the in far-field light microscopy sets a resolution limit of ;200 basis for photoactivated localization microscopy (25), fluo- nm in the focal plane and 500 nm in the axial direction (1,2). rescence photoactivation localization microscopy (26), and Ways to break this resolution limit include reducing the ob- stochastic optical reconstruction microscopy (27). Use of servation volume or, correspondingly, increasing the avail- these methods has been largely restricted to fixed samples, able Fourier space of the image, as elegantly demonstrated however, because of limits set by photobleaching of cur- by 4Pi (3,4), patterned illumination (5,6), reversible satura- rently available fluorophores (28). For accurate determina- ble optical fluorescent transition (RESOLFT) (7), and stim- tion of axial position within ;200 nm of the specimen ulated emission depletion microscopy (STED) (8–10). These surface, TIRF is particularly efficient, because of the pene- methods illuminate ensembles of fluorescent molecules and tration fall-off of the evanescent field (29,30). This property can achieve resolutions as low as 20 nm in the focal plane has been used to demonstrate the inward movement of and 100 nm in the axial direction. To date, only 4Pi and clathrin-containing structures at the plasma membrane by STED have been successfully used to image living cells with means of alternating TIRF and WF illumination (15,16). It is 100- to 150-nm resolution (9,11), whereas use of all of the possible to determine experimentally the distance depen- other methods has been restricted to fixed samples due, in dence of the evanescent field and to track the axial position part, to high excitation intensities that result in photo- (determined as emitted intensity) of a single fluorophore with bleaching and phototoxicity (12). nanometer precision using just 1000 photons—a method Diffraction-limited multiprotein structures, such as clath- referred to as evanescence nanometry (31). rin coated pits (13–16), focal adhesion sites (17–19), and In the approach described here, of general application to budding viruses (20–22), form on the plasma membrane freely rotating fluorescent dipoles, we have taken advantage of cells. Current applications of live-cell imaging methods, of evanescence nanometry and independent detection of two such as confocal, wide-field (WF), and total internal reflec- fluorophores to track simultaneously the axial positions of tion fluorescence (TIRF) microscopies, can be used to follow the fluorophore centroids within a diffraction-limited object the assembly dynamics of such structures, including the on the surface of a living cell. We use WF images to correct independent recruitment of differentially labeled compo- for the varying number of molecules within the object as nents. Because the centroid of a fluorophore can be located it assembles or disassembles, and the ratio of TIRF to with far more accuracy than the nominal resolution, it is WF intensity to determine position. With this method, which theoretically possible to ‘‘resolve’’ two components to an we call differential evanescence nanometry (DiNa), we accuracy of ,10 nm, provided that ;10,000 photons/ can determine the difference in axial positions of the two fluorophore centroids to an accuracy of ;10 nm. The posi- tion of one fluorophore serves as a reference for the other, Submitted July 12, 2007, and accepted for publication October 24, 2007. thus correcting for overall axial motions of the cell surface. Address reprint requests to Tomas Kirchhausen, Dept. of Cell Biology and Clathrin-coated pits and coated vesicles form at the plasma Immune Disease Institute, Harvard Medical School, 200 Longwood Ave., Boston, MA 02115. Tel.: 617-278-3140; Fax: 617-278-3125. E-mail: membrane of eukaryotic cells. The assembly dynamics of [email protected]. these diffraction-limited objects has been followed in cells Editor: David W. Piston. Ó 2008 by the Biophysical Society 0006-3495/08/03/2333/10 $2.00 doi: 10.1529/biophysj.107.117234 2334 Saffarian and Kirchhausen expressing fluorescent chimeras of clathrin (13–16) and its in which the superscripts r and g stand for the red and green AP-2 adaptor complex (13). BSC1 monkey cells are partic- channel quantities, respectively. ularly advantageous for such studies, since .95% of the clathrin-coated pits on the plasma membrane are diffraction- Normalization of the excitation intensity and limited and barely move in the focal plane during their as- establishment of origin sembly (13). The growth period of the coated pit has an average lifetime of 50 s and results in a fully formed coated Absolute position measurements with respect to the glass vesicle upon scission from the plasma membrane. We de- require the knowledge of the exact WF and TIR excitation scribe here the use of DiNa to monitor the axial separation intensities at each point in the image, as shown in Eq. 5. TIR between clathrin and AP-2 as the coat forms in the cell. Our calibration measurements as outlined in this article measure observations clearly indicate an increasing separation of these the penetration depth of the TIR but do not measure the ab- components of up to ;30 nm, reached by the end of the solute laser intensities. Thus, a change in the position of mol- assembly process. This separation in the average distributions ecules can be measured, but their absolute position will not be of clathrin and AP-2 agrees with the relative asymmetric known. By normalizing the fluorescence intensities to inten- positions of clathrin and AP-2 within coated vesicles isolated sities at a particular location, a reference point can be estab- ¼ from calf brains, as determined by cryoelectron microscopy lished. At this position, which has z 0, the fluorescence of tomographic reconstruction from single coated vesicles (32). the TIRF and WF signals are equal (by the normalization step). If the number of contributing molecules in the object is fixed, then the WF intensity is constant; under these condi- THEORY tions, a point of origin can be found anywhere by normalizing When a laser beam is reflected at an interface between two the TIRF signal to its WF signal. This normalization should be media, the total internal reflection creates an evanescent carried out in both channels at the same time, so that in that par- field, which decays exponentially from the interface: ticular point, the separation of colors would be zero by default. When the object grows by addition of molecules until it 0 z ITIRFðzÞ¼I Exp À ; (1) reaches a final size, there may not be sufficient molecules at TIRF d the beginning of the structure formation to perform a where ITIRF(z) is the excitation intensity in the evanescence normalization. Normalizing the intensities at the beginning 0 wave and ITIRF is the excitation intensity directly adjacent of such a trace has advantages, however, because the overall to the interface surface. The total TIRF from N molecules size of the structure is very small at the beginning, and positioned at z can be written as therefore it can be assumed that the two colors truly overlap 0 z at this point. To normalize the intensities in such experi- FTIRF ¼ NqITIRFðzÞ¼NqI Exp À ; (2) TIRF d ments, the fluorescence intensities are added during an initial in which q is a product of the quantum yield, the absorption segment of structure formation (,30% of the structure), and coefficient, and the detection efficiency. The total fluores- their ratio is used for normalization as shown below: R = cence under wide-field illumination, FWF, can be written as 1 3 F ðtÞdt I0 a ¼ R 0 WF WF : ¼ ; 1=3 0 (7) FWF NqIWF (3) ð Þ I 0 FTIRF t dt TIRF where I is independent of z. The axial position of the WF The integration of signal allows for a reasonable signal/noise group of molecules can be calculated as ratio while normalizing the fluorescence values. There are two I F assumptions made in Eq. 7. We assume that the structure does z ¼d 3 log WF TIRF : (4) 0 not have a significant size when ,30% of the structure is com- ITIRFFWF pleted; in addition, we assume that the building process pro- If the number of molecules is a function of time, the above ceeds relatively quickly, and that in the initial phase, the relationship will still work, as long as the TIRF and the WF structure does not move appreciably in the vertical direction.
Details
-
File Typepdf
-
Upload Time-
-
Content LanguagesEnglish
-
Upload UserAnonymous/Not logged-in
-
File Pages10 Page
-
File Size-