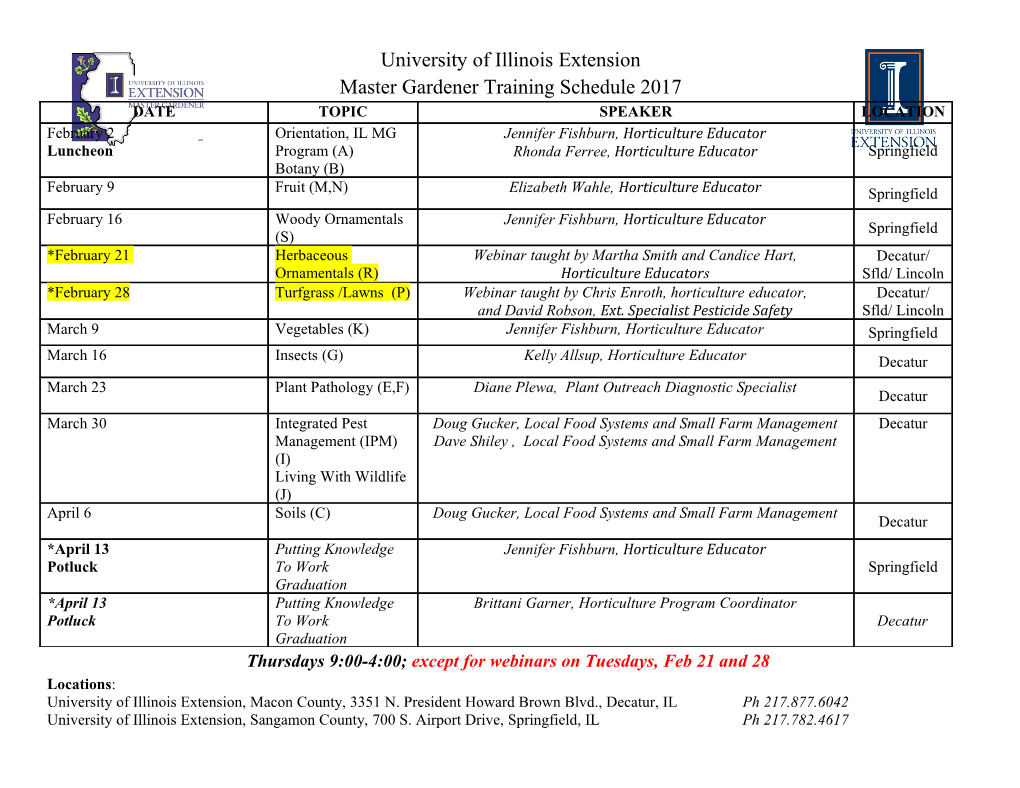
UNITED STATES DEPARTMENT OF THE INTERIOR GEOLOGICAL SURVEY 40Ar/38Ar Age-Spectrum Data for Rocks of the Bronson Hill Terrane, Central New England by Julie L. Boyd1, Michael J. Kunk2, and Robert P. Wintsch3 Open-File Report 94-126 January 1994 This report is preliminary and has not been edited or reviewed for conformity with U.S. Geological Survey editorial standards and stratigraphic nomenclature. Any use of trade names is for descriptive purposes only and does not imply endorsement by the U.S.G.S. 1 U.S. Geological Survey, MS 981, Reston, Virginia 22092, and Department of Geological Sciences, Indiana University, Bloomington, Indiana 47405 2 U.S. Geological Survey, MS 981, Reston, Virginia 22092 3 Department of Geological Sciences, Indiana University, Bloomington, Indiana 47405 FROK BEST WASLABLE CCflf TABLE OF CONTENTS INTRODUCTION 3 SAMPLING STRATEGY 3 METHODS 5 Sample Preparation 5 Sample Analysis 5 Isotopic Data Reduction 6 SAMPLE DESCRIPTIONS 8 RESULTS 20 Condensed Tabular Argon Data 22 *Ar/*Ar Data 24 ACKNOWLEDGEMENTS 126 REFERENCES 126 INTRODUCTION The purpose of this report is to present 40Ar/38Ar data from hornblende, biotite, and muscovite separated from metamorphic rocks collected from the Bronson Hill terrane in central New England (Fig. 1). This data was collected to characterize the metamorphic/cooling history of the Bronson Hill terrane. In the central part of the New England Appalachians the Bronson Hill terrane lies close to the Acadian metamorphic high (Robinson, et al., 1986), but to the south the effects of Alleghanian metamorphism become stronger Brookins, 1970; (Brookins and Armstrong, 1980; Pressel and Armstrong, 1980; Wintsch and Sutter, 1986). The scientific goal of this study is to characterize the transition between the northern Acadian and southern Alleghanian orogenic events in the Bronson Hill terrane. Ultimately, the comparison of the metamorphic cooling history of the Bronson Hill terrane with that of adjacent terranes will allow for a better understanding of the regional tectonothermal evolution of this portion of New England and may reveal some history of terrane assembly. The primary approach used in this research is 40Ar/38Ar mineral age dating (thermochronology). This method monitors the time-temperature history preserved in mineral isotopic compositions, and is especially useful in constraining the times of cooling through closure (Dodson, 1973) from peak metamorphic conditions. The results of our analysis are presented in this report; a preliminary geological interpretation of these results appear in Boyd, et al. (1993) and Wintsch, et al. (1993a;b). SAMPLING STRATEGY To characterize the transition between the metamorphic histories of Acadian and Alleghanian orogenic events in the Bronson Hill, samples were collected along a north-south traverse (Fig. 1). The northern limit of sampling was the southern Pelham dome which connects our data to that of Spear and Harrison (1989), and Tucker and Robsinson (1990; 1993) in northcentral Massachusetts and adjacent New Hampshire (Harrison et al. 1989). Samples were collected at 5-10 km intervals from the southern Pelham dome south to Long Island Sound. Amphibolites, granodioritic gneisses, muscovite schists, and pegmatites were collected, and hornblende, muscovite, and biotite were separated. Pelham Dome Massachusetts I / Connecticut i_ LEGEND Willimantic O This Study ° Tucker & Robinson 1990 Glaston Window $ Spear & Harrison 1989 Dome + Harrison et. al, .1989 A Brookins & Armstrong 1980 v Brookins 1970 Wintsch & Sutter 1986 X Pressel & Armstrong 1980 1-95 New Haven * Figure 1. Location map of samples dated. Includes locations of samples not dated in this study (see Legend). The light gray represents the Bronson Hill terrane and the dark gray denotes the Avalon terrane. METHODS Sample Preparation All samples were crushed, ground, and sieved to >250, 180, 150, 125, and <125 pm sizes. The largest size fraction that excluded most composite grains was selected for mineral separation by heavy liquids, magnetic separation and/or paper shaking. Composite grains were eliminated by ultrasonic abrasion where necessary. Purity to >99% of muscovite and biotite and 99.9% for amphibole was checked with a binocular microscope using reflected light. Approximately 1000 mg. of amphibole, 100 mg. of muscovite, and 50- 100 mg. of biotite were packaged in aluminum capsules and sealed under vacuum in quartz tubes. Samples were then irradiated in the central thimble facility at the TRIGA reactor (GSTR) at the U.S. Geological Survey, Denver, Colorado. The monitor mineral was MMHb-1 hornblende which has an age of 519.4 ± 2.5 Ma (Alexander et al., 1978; Dalrymple et al., 1981). The type of container, and the geometry of samples and standards is similar to that described by Snee et al. (1988). Sample Analysis All samples were analyzed on a VG Isotopes, Ltd., Model 1200 B Mass Spectrometer at the U.S. Geological Survey, Reston, using the step heating method. Heating for 10 minutes per step followed a schedule of 8-15 steps per sample. The number and temperature of heating steps was selected to limit the percentage of gas released to less than 20%/step for most samples. Heating of all samples was done in a small volume molybdenum-lined "low blank" tantalum furnace. Temperature was monitored by a W5Re- W^Re thermocouple and controlled by a proportional, programmable controller. The furnace and the rear manifold were evacuated as necessary by a turbo molecular pump. Two isolated ion pumps evacuated the front manifold and the mass spectrometer tube. During normal operation, the gas to be analyzed was purified in the rear manifold by a Saes ST707 Zr-V-Fe getter operated at room temperature. Gas was equilibrated with the front manifold with an empty cold finger (in the rear manifold) chilled with liquid N2 to remove condensables (chiefly water), and cleaned in the front manifold by a Saes ST101 Al-Zr getter operated at 400°C and a Ti (H2) getter operated at a constant 350°C. An activated charcoal finger submerged in a constant boiling mixture of dry ice and acetone was used to remove gasses with a molecular weight greater than 60 or 80 (primarily noble gases) prior to the admission of the argon dominated gas to the mass spectrometer by expansion. The argon-rich gas was further purified in the mass spectrometer by a second Saes ST101 getter operated at room temperature. Its successful operation could be monitored by the drop in counts of mass 44 (dominated by GO2) after the first gas analysis cycle. All phases of the analysis; heating, gettering, equilibration, and mass spectrometer analysis were performed under computer control. Argon isotopes with masses 40 through 36 and GO2, mass 44 were analyzed as a function of time in five analysis cycles. 40Ar, MAr, and ^Ar peaks and their baselines, were measured as a series of four, five second integrations in each of the five cycles, that were then averaged. ^Ar and 37Ar peaks, and their baselines, were measured for only one, five second integration per cycle. After the analysis, the mass spectrometer was evacuated. If necessary, the fraction of gas remaining in the front manifold could be introduced into the mass spectrometer for a replicate "split" analysis, but with a signal 3.6 X smaller (see tables below). Isotopic Data Reduction All the Ar isotopic data were reduced using an updated version of the computer program ArAr* (Haugerud and Kunk, 1988) and decay constants recommended by Steiger and Jager (1977). The isotopic measurements made in the five cycle analysis had baseline values subtracted and then were regressed, to time zero, using standard linear regression techniques. These regressed values and associated statistical estimates of analytical uncertainties of the time zero peak values were used in the data reduction. No corrections for furnace blanks were made because blanks were routinely less than 0.1% of sample signal at all temperatures. Corrections for interfering reactor-produced argon isotopes from Ga, K, and Gl in the sample were made using the production ratios given in Dalrymple et al. (1981). Errors included in calculating ages or ratios include analytical errors in the analysis, decay factor uncertainties, measured atmospheric or calculated initial ^Ar/^Ar ratios, the irradiation parameter J, the production ratios of the various reactor induced argon producing reactions, the inital ^Ar/^Ar ratio, and the age of the monitor (Haugerud and Kunk, 1988). In this project unique variables arise from the neutron flux as revealed through the calculated J values using Minnesota hornblende MMHb-1 (Alexander et al., 1978) and its age of 519.4 Ma ± 0.5%. Over 20 hornblende monitors were added to a single vial, and these defined a smooth curve where J varied from 0.0088 to 0.0098 across the six, 12 cm. long vials. Monitors were added to the top and/or bottom of each of the other five vials to assess any rotational fluctuation in the 6-vial package; none was found, within the limits of analytical precision. Sensitivities in moles ^Ar/count were calculated from analytical results on Minnesota hornblende MMHb-1 and its published composition (Alexander et al., 1978), and these sensitivity values are included in the data table for each sample. The tables and figures below include the identification of plateau ages, isochron ages, minimum ages, fusion ages and total gas ages. Plateau ages include two or more contiguous steps that overlap within experimental error, and whose cumulative MArK comprises greater than 50% of the total potassium derived MAr (Fleck et al., 1977; Snee et al., 1988). For all samples analyzed by the ^Ar/^Ar age spectrum dating technique, all steps were examined for colinearity on isotope correlation diagrams to assess if non-atmospheric argon components were trapped in any samples and to calculate an isochron age. In several samples points that were deemed to be not colinear were deleted from the isotope correlation diagram.
Details
-
File Typepdf
-
Upload Time-
-
Content LanguagesEnglish
-
Upload UserAnonymous/Not logged-in
-
File Pages129 Page
-
File Size-