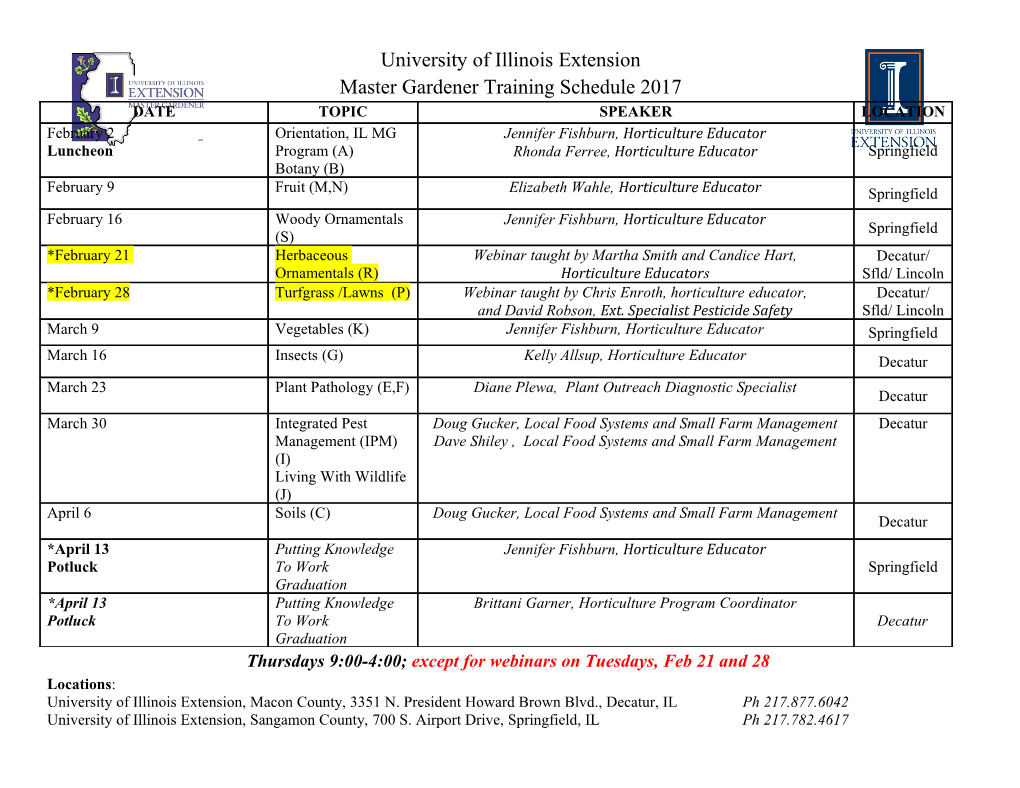
Click Here for Full Article WATER VAPOR AND THE DYNAMICS OF CLIMATE CHANGES Tapio Schneider,1 Paul A. O’Gorman,2 and Xavier J. Levine1 Received 29 June 2009; accepted 3 December 2009; published 2 July 2010. [1] Water vapor is not only Earth’s dominant greenhouse culation and of zonally asymmetric tropical circulations, as gas. Through the release of latent heat when it condenses, well as the kinetic energy of extratropical baroclinic eddies, it also plays an active role in dynamic processes that shape can be lower than they presently are both in much warmer the global circulation of the atmosphere and thus climate. climates and in much colder climates. We discuss how latent Here we present an overview of how latent heat release heat release is implicated in such circulation changes, partic- affects atmosphere dynamics in a broad range of climates, ularly through its effect on the atmospheric static stability, ranging from extremely cold to extremely warm. Contrary and we illustrate the circulation changes through simulations to widely held beliefs, atmospheric circulation statistics with an idealized general circulation model. This allows us can change nonmonotonically with global‐mean surface to explore a continuum of climates, to constrain macro- temperature, in part because of dynamic effects of water scopic laws governing this climatic continuum, and to place vapor. For example, the strengths of the tropical Hadley cir- past and possible future climate changes in a broader context. Citation: Schneider, T., P. A. O’Gorman, and X. J. Levine (2010), Water vapor and the dynamics of climate changes, Rev. Geophys., 48, RG3001, doi:10.1029/2009RG000302. 1. INTRODUCTION 2007; Johanson and Fu, 2009]. This widening of the Had- ley circulation is often linked to the decrease in the moist [2] Water vapor is not only important for Earth’s radiative balance as the dominant greenhouse gas of the atmosphere. adiabatic temperature lapse rate with increasing surface It is also an active player in dynamic processes that shape temperature, which results in an increased tropical static the global circulation of the atmosphere and thus climate. stability and can lead to a widening of the Hadley circula- The latent heat released when atmospheric water vapor tion, at least in dry atmospheres [e.g., Held, 2000; Walker condenses and the cooling of air through evaporation or and Schneider, 2006; Frierson et al., 2007b; Korty and sublimation of condensate affect atmospheric circulations. Schneider, 2008]. Yet it is unclear how the width of the Although the mechanisms are not well understood, it is Hadley circulation in an atmosphere in which water vapor is widely appreciated that heating and cooling of air through dynamically active relates to the static stability or, in fact, phase changes of water are integral to moist convection and how the static stability thought to be relevant (that at the dynamics in the equatorial region. But the fact that water subtropical termini of the Hadley circulation) is controlled. vapor plays an active and important role in dynamics [3] Here we present an overview of dynamic effects of globally is less widely appreciated, and how it does so is water vapor in the global circulation of the atmosphere and only beginning to be investigated. For instance, there is in climate changes. What may be called water vapor kine- — evidence that the width of the Hadley circulation has matics the study of the distribution of water vapor given — increased over the past decades [e.g., Hu and Fu, 2007; the motions of the atmosphere has recently been reviewed Seidel and Randel, 2007; Seidel et al., 2008], and it also by Held and Soden [2000], Pierrehumbert et al. [2007], and increases in many simulations of climate change in response Sherwood et al. [2010]. We bracket off questions of water to increased concentrations of greenhouse gases [e.g., vapor kinematics to the extent possible and instead focus on — Kushner et al., 2001; Lu et al.,2007;Previdi and Liepert, what may be called water vapor dynamics the study of the dynamic effects of heating and cooling of air through phase changes of water. 1California Institute of Technology, Pasadena, California, USA. 2Department of Earth, Atmospheric and Planetary Sciences, [4] Our emphasis lies on large scales, from the scales of Massachusetts Institute of Technology, Cambridge, Massachusetts, USA. extratropical storms (∼1000 km) to the planetary scale of the Hadley circulation. In motions on such large scales, the Copyright 2010 by the American Geophysical Union. Reviews of Geophysics, 48, RG3001 / 2010 1of22 8755‐1209/10/2009RG000302 Paper number 2009RG000302 RG3001 RG3001 Schneider et al.: WATER VAPOR AND CLIMATE CHANGE RG3001 release of latent heat through condensation generally is more vaporization. Consistently but unlike what would occur in important than the cooling of air through evaporation or the real world, ice is ignored in the model, be it as cloud ice, sublimation of condensate: the residence times of vapor and sea ice, or land ice. We obtained a broad range of statistically condensate are similar (days and longer), and so are the steady, axisymmetric, and hemispherically symmetric cli- specific latent heat of vaporization and that of sublima- mates by varying the optical thickness of an idealized atmo- tion, but the atmosphere in the global mean contains about spheric longwave absorber, keeping shortwave absorption 250 times more water vapor (∼25 kg m−2) than liquid water fixed and assuming gray radiative transfer. The climates and ice (∼0.1 kg m−2)[Trenberth and Smith, 2005]. None- have global‐mean surface temperatures ranging from 259 K theless, even motions on large scales are affected by smaller‐ (pole‐equator surface temperature contrast 70 K) to 316 K scale dynamics such as moist convection, for which cooling (temperature contrast 24 K) and atmospheric water vapor through evaporation of condensate and the convective concentrations varying by almost 2 orders of magnitude. We downdrafts thereby induced are essential [e.g., Emanuel et will discuss dynamic effects of water vapor in past and al., 1994]. The emphasis on large scales allows us to side- possible future climates in the context of this broad sample line some of the complexities of moist convection and from a climatic continuum, making connections to observa- consider only the collective effect of many convective cells tions and more comprehensive GCMs wherever possible. on their large‐scale environment, assuming that the con- This allows us to examine critically, and ultimately to reject, vective cells adjust rapidly to their environment and so are some widely held beliefs, such as that the Hadley circulation in statistical equilibrium (“quasi‐equilibrium”) with it would generally become weaker as the climate warms or [Arakawa and Schubert, 1974]. Our reasoning about the that extratropical storms would generally be stronger than effect of moist convection on large‐scale motions builds they are today in a climate like that of the LGM with larger upon the cornerstone of convective quasi‐equilibrium pole‐equator surface temperature contrasts. dynamics, well supported by observations and simulations [7] Section 2 reviews energetic constraints on the con- of radiative‐convective equilibrium: convection, where it centration of atmospheric water vapor and precipitation as occurs, tends to establish a thermal stratification with moist background for the discussion of how water vapor dynamics adiabatic temperature lapse rates (see Emanuel et al. [1994], affects climate changes. Section 3 examines tropical circu- Emanuel [2007], and Neelin et al. [2008] for overviews). lations, with emphasis on the Hadley circulation. Section 4 [5] Dynamic effects of water vapor in the global circula- examines extratropical circulations, with emphasis on tion of the atmosphere have typically been discussed in the extratropical storms and the static stability, which occupies a context of specific past climates, such as that of the Last central place if one wants to understand the effects of water Glacial Maximum (LGM), or possible future climate chan- vapor on extratropical dynamics. Section 5 summarizes ges in response to increased concentrations of greenhouse conclusions and open questions. gases. We view past and possible future climates as parts of a climatic continuum that is governed by universal, albeit largely unknown, macroscopic laws. Our goal is to constrain 2. ENERGETIC CONSTRAINTS ON WATER VAPOR the forms such macroscopic laws may take. They cannot be CONCENTRATION AND PRECIPITATION inferred from observational data as it can be misleading to [8] Water vapor dynamics is more important in warmer infer laws governing climate changes from fluctuations than in colder climates because the atmospheric water vapor within the present climate (e.g., from El Niño and the concentration generally increases with surface temperature. Southern Oscillation, as we will discuss further in section 3). This is a consequence of the rapid increase of the saturation And they are difficult to infer from simulations with com- vapor pressure with temperature. According to the Clausius‐ prehensive climate models, whose complexity can obscure Clapeyron relation, a small change dT in temperature T leads the chain of causes and effects in climate changes. to a fractional change de*/e* in saturation vapor pressure e*of [6] Therefore, we illustrate theoretical developments in e* L what follows with simulations of a broad range of climates T; ð1Þ e* R T 2 with an idealized general circulation model (GCM). The v simulations are described in detail by O’Gorman and where Rv is the gas constant of water vapor and L is the Schneider [2008a]. They are made with a GCM similar to specific latent heat of vaporization. If one substitutes tem- that of Frierson et al. [2006], containing idealized peratures representative of near‐surface air in the present representations of dynamic effects of water vapor but not climate, the fractional increase in saturation vapor pressure accounting for complexities not directly related to water with temperature is about 6–7% K−1; that is, the saturation vapor dynamics.
Details
-
File Typepdf
-
Upload Time-
-
Content LanguagesEnglish
-
Upload UserAnonymous/Not logged-in
-
File Pages22 Page
-
File Size-