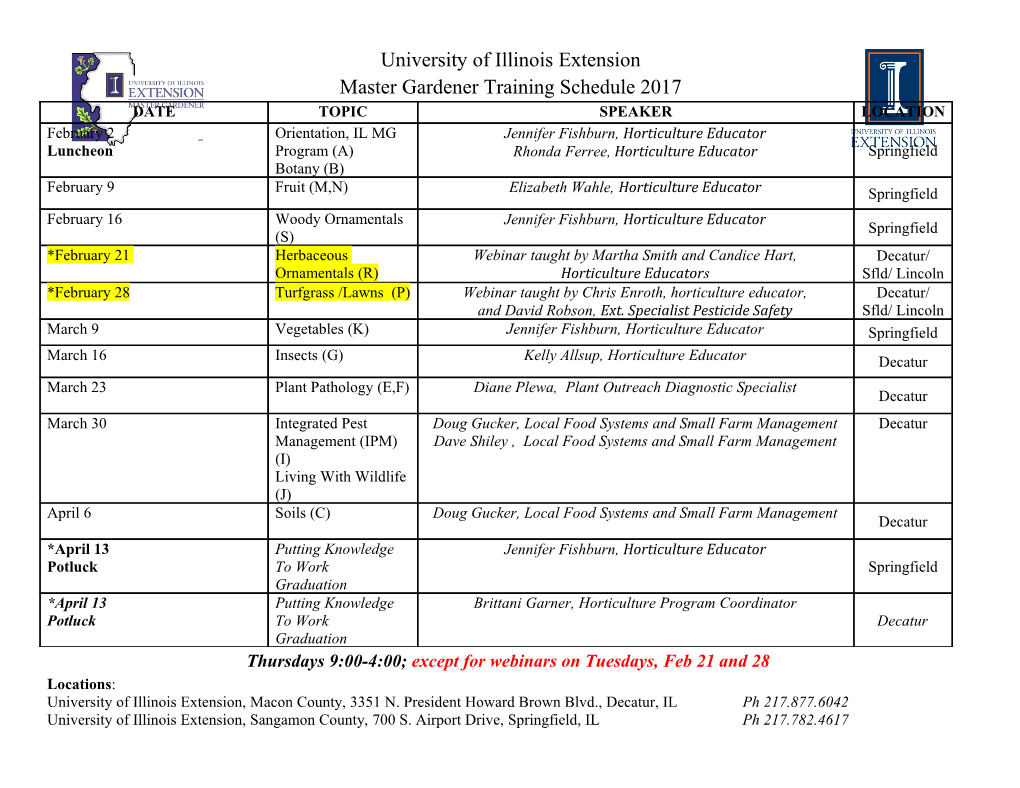
129 C hapter 5 Quantization of Matter Photo: Brown University An experiment showing the path of single electrons passing through liquid helium. Atoms of the different chemical elements are different aggregations of atoms [particles] of the same kind.... Thus on this view we have in the cathode rays matter in a new state, a state in which the subdivision of matter is carried very much further than in the ordinary gaseous state: a state in which all matter—that is, matter derived from different sources such as hydrogen, oxygen, etc.—is of one and the same kind; this matter being the substance from which all the chemical elements are built up. J. J. THOMSON , Philosophical Magazine 44, 293 (1897) Introduction The study of Einstein’s special theory of relativity has expanded and com - pletely altered our fundamental view of nature from that suggested by classical mechanics. It is important to realize that our new perception of the concepts of length, mass, time, and energy resulted from essentially 130 Ch. 5 Quantization of Matter one new basic postulate of nature (the invariance of the speed of light) and an application of classical mechanics to fundamental physical consid - erations of macroscopic phenomena. Additional deviations from classical physics and insights of microscopic phenomena will be detailed in this and the next few chapters, as we consider other theoretical and experimental contributions to modern physics. The method of inquiry is similar to that utilized in the study of Einsteinian relativity, in that a few new fundamen - tal postulates of nature are combined with well known principles of clas - sical mechanics and electromagnetic theory to produce a new nonclassical view of nature on the microscopic level. The immediate objective of this chapter is to study the quantization of matter, a concept that suggests matter is composed of basic constituents or minute particles. After a brief review of the evolution and scientific ac - ceptance of this atomic view , the qualitative physical properties of an elec - tron will be investigated. This is immediately followed by a study of the early measurements and estimates of the specific charge ( e/me), absolute charge, mass, and size of an electron. The emphasis of these discussions is not on the actual experiments and analyses performed by physicists in obtaining these early estimates. Instead, the logical application of basic principles of classical physics is emphasized in the development of rela - tionships capable of predicting theses fundamental physical properties of an electron. Further, a limited discussion of the modern model of the atom and nucleus is presented, followed by theoretical considerations for the mass, size, and binding energy of an atom. As a number of fundamental relationships of classical electromagnetic theory will be utilized in this chapter, a review of the basic equations, SI units, defined units, and con - ventional symbols presented in a general physics textbook might prove beneficial. 5.1 historical perspective The concept of matter being quantized (i.e., discrete) was suggested as early as the fifth century B.C. by Greek philosopher Democritus. This view, however, was mostly disregarded for nearly two thousand years by scien - tists in favor of the Aristotelian philosophy that consider space and matter as being continuous . Serious theoretical support for the atomic view of matter by Pierre Gassendi, Robert Hooke, and Isaac Newton appeared in the middle and latter part of the seventeenth century. These efforts, how - ever, were essentially ignored for nearly another hundred years, before ini - tial experimental evidence from quantitative chemistry was available in support of the quantization of matter . Of the many scientists involved in the development of quantitative 5.1 Historical Perspective 131 chemistry at the turn of the nineteenth century, the more noteworthy in - clude chemists Antoine Lavoisier, J. L. Proust, John Dalton, J. L. Gay- Lussac, and the Italian physicist Amedeo Avogadro. The work of these individuals clearly established that basic substances participate in chemical reactions in discrete or quantized entities. Their efforts led to the definition of chemical elements and the concept of atomic masses (originally called atomic weights ). In fact Dalton suggested each element was composed of physically and chemically identically atoms and that these atoms were dif - ferent from the atoms of any other element. He also introduced the con - cept of atomic masses; however, it was Avogadro who provided the best rationale for finding atomic masses by way of his hypothesis that at the same temperature and pressure equal volumes of gases contain the same number of particles . He was also the first to recognize that two or more atoms could combine to form what he called a molecule , a concept that was not fully understood until the development of quantum mechanics in the twentieth century. His hypothesis is of fundamental importance to physics and physical chemistry in that it predicts the number of atoms or molecules in one mole of a substance (any element or compound) as being exactly equal to a number No, called Avogadro’s constant. Although the absolute magnitude of No was not known for more than fifty years after Avogadro’s hypothesis, knowledge of its existence was sufficient and of primary importance in the development of relative atomic masses for the chemical elements. In the last section of this chapter Avogadro’s hypoth - esis and the value of No will be utilized in calculating the absolute mass and size of an atom. An enormous amount of evidence for the quantization of matter was provided by the advent and development of kinetic theory in the nine - teenth century, which was complementary to and independent of the view suggested by quantitative chemistry. Kinetic theory arises from the appli - cation of Newtonian mechanics to a gas considered as a system consisting of a very large number of identical particles. These particles are imagined to exist in a state of random motion and have elastic collisions with one another and the gas container. This large and very elegant subject was the first microscopic model of matter describing the physical properties of a gas. It was initially developed in part by Daniel Bernoulli In 1738; however, the major contributions and development occurred in the nineteenth cen - tury and were brought about notably by J. P. Joule, R. J. Clausius, J. C. Maxwell, L. Boltzmann, and J. W. Gibbs. Although kinetic theory per se is not germane to our immediate objectives, it is appropriate to acknowl - edge its contribution to the atomic view of nature. Many of the results of kinetic theory will be independently developed and discussed later in this textbook, when we consider the fundamental principles and physical ap - plications of statistical mechanics . 132 Ch. 5 Quantization of Matter One other contribution supporting the atomic view of matter came from the law of electrolysis developed by Michael Faraday in 1833. By al - lowing electricity to flow though electrolytic solutions and observing the components of the solution being liberated at the electrodes, Faraday was able to predict the existence of a discrete unit of electrical charge. His work supported not only the quantization of matter, but also the quantization of electrical charge . This discreteness in nature was later confirmed by ex - perimental investigation of cathode and canal rays, which led to measure - ments of the elemental electrical charge in nature and measurements of atomic masses, respectively. The qualitative physical properties of cathode rays is the topic of discussion in the next section, while canal rays will be considered in some detail in Section 5.6. 5.2 Cathode rays During the second half of the nineteenth century considerable scientific effort was devoted to the investigation of electrical discharge through rar - efied gases. In 1853 a Frenchman by the name of Masson discharged an electrical spark through a rarefied gas and found that the glass tube con - taining the gas was filled with a bright glow, instead of the normal spark as observed in air. A few years later the German glass blower Heinrich Geissler manufactured a number of these gaseous discharge tubes and sold them to scientists around the world. The Geissler tube , as illustrat ed in Figure 5.1, essentially contained an anode and cathod e electrode em - bedded in a partially evacuated glass tube. As the in ternal pressure to the tube is further decreased, the electrical discharge through the rarefied gas undergoes a number of different phases, as was reported by W. Crookes, Faraday, and others. At a pressure of roughly 0.01 m m o f H g a g l ow dis - charge is produced, as the entire tube tends to glow w it h a f a int g reenish light. The initial explanation was that invisible rays, c a lled cathode rays , emanating from the cathode electrode would strike the walls of the tube and cause a florescence of the glass. The existence of these invisible cath - ode rays caused considerable investigatory excitement in the scientific com - munity during the remainder of the nineteenth century. Figure 5.1 Discharge tube A simple Geissler dis - charge tube containing two electrodes. Anode Cathode 5.2 Cathode Rays 133 Cathode rays Cathode Figure 5.2 A Crookes demonstra - tion tube illustrating Object the rectilinear propaga - Shadow tion of cathode rays. Anode It can be easily demonstrated that the rays travel from the cathode to the anode in a straight line by using a discharge tube similar to that of Figure 5.2, where the dashed lines represent the rays emanating from a point source . A greenish fluorescence is observed where the rays strike the glass, while the glass in the shadow of the object remains dark. Since the shadow is distinctive and always on the side opposite the cathode, the rays must be traveling in straight lines and emanating from the cathode elec - trode.
Details
-
File Typepdf
-
Upload Time-
-
Content LanguagesEnglish
-
Upload UserAnonymous/Not logged-in
-
File Pages50 Page
-
File Size-