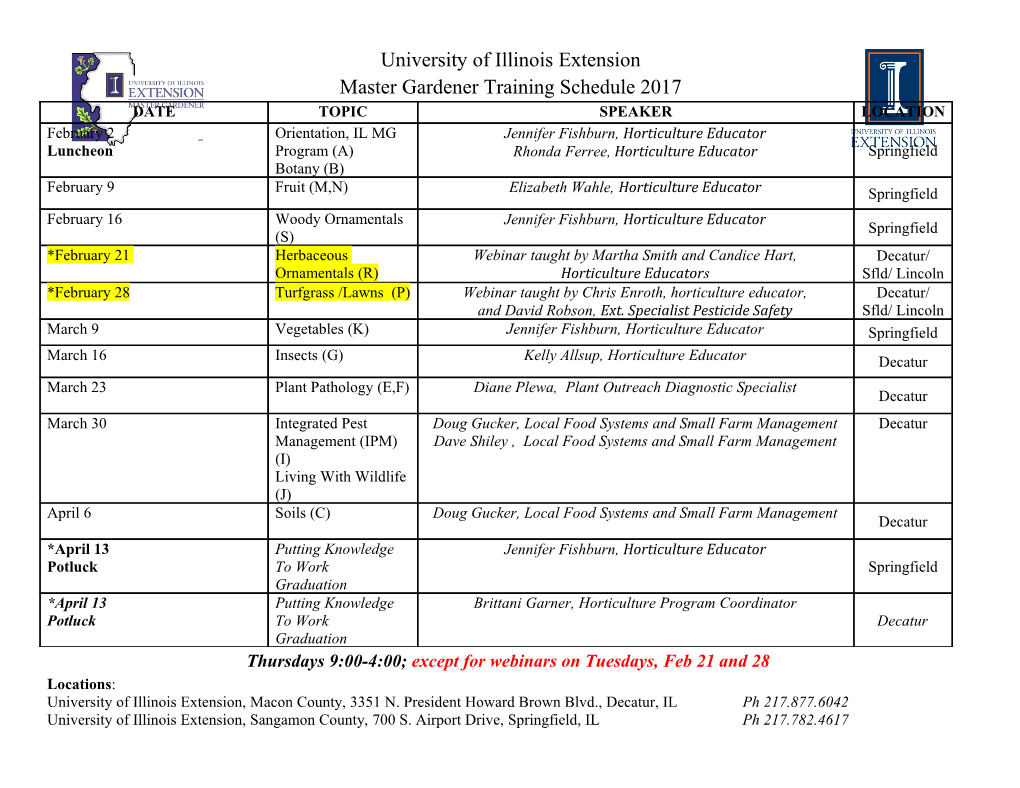
Neuronal Signaling Neuroscience Fundamentals > The Nerve Cell > The Nerve Cell NEURONAL SIGNALING SUMMARY ACTION POTENTIALS See: Action Potentials Key Features • All-or-nothing events • Self-propagating • Conduction speed determined by axon diameter (thicker means faster) and amount of myelination (more myelin means faster transmission). CHEMICAL SYNAPSE Steps of Chemical Synapsis 1) The action potential travels down axon of presynaptic neuron. 2) Vesicle fuses with plasma membrane and releases neurotransmitter into the synaptic cleft. 3) Neurotransmitters bind to ligand-gated ion channels, opening them. 4) Ions entering through the open channels cause a depolarization of the membrane, opening voltage-gated ion channels. 5) Ions pass through these voltage-gated ion channels, causing further depolarization of the membrane. 6) This depolarization (action potential) travels along the membrane as more voltage-gated ion channels are opened. ELECTRICAL SYNAPSE • Cytoplasms of adjacent neurons are connected, allowing ions (and action potentials) to travel directly to the next cell. NEURONAL CODING OF STIMULUS STRENGTH Action Potential Frequency • Stimulus strength is based on frequency of action potentials. Action Potential Strength • Strong stimuli can produce another action potential during the relative refractory period. 1 / 7 ABSOLUTE REFRACTORY PERIOD No further action potentials • Voltage-gated sodium channels are open or inactivated so another stimulus, no matter its strength, CANNOT elicit another action potential. RELATIVE REFRACTORY PERIOD Some capability of producing action potential • Voltage-gated sodium channels have mostly reset and some voltage-gated potassium channels are still open. • Large stimuli are capable of producing an action potential during this period. MYELINATION & CONDUCTION SPEED Degree of myelination affects action potential conduction speed • Bare plasma membrane - After stimulus, voltage quickly degrades as ions leak across the membrane • Non-myelinated axon - While voltage still quickly degrades, the action potential is regenerated as new voltage-gated ion channels are opened; conduction speed is slow because it takes time to open new channels • Myelinated axon - Due to myelin insulating the membrane, voltage does not degrade as quickly; new voltage-gated ion channels are only opened at the Nodes of Ranvier so the conduction speed is faster (because the action potential appears to "jump" from Node to Node, it is called saltatory conduction). GASSER CLASSIFICATION OF NERVE FIBERS Groups A, B, C • Group A – Largest diameter, thick myelin sheaths, conduction speeds of up to 120m/s • Group B – Intermediate diameter, lightly myelinated, conduction speeds of around 10m/s • Group C – Smallest diameters, not myelinated, conduction speeds of 1m/s or less FULL-LENGTH TEXT OVERVIEW 2 / 7 • Here we will learn about how a signal is received by a neuron and transmitted to another neuron. • First, start a table to list some key concepts of neuronal signaling. • Denote that neuronal action potentials are all-or-nothing events; they either fully happen or don't happen at all, like firing a gun. - There are no partial action potentials. - They are also self-propagating. Once started, they continue to happen along the length of an axon (like a domino effect). • Denote that action potential conduction velocity depends on two factors: - Axon diameter, where larger usually results in faster speed. - And amount of myelination, where more myelination results in faster speed (we will discuss this concept in more detail shortly). CHEMICAL SYNAPSE Key Structures First, let's explore how signals are transmitted across chemical synapses (the more common type) to start an action potential on the next cell. • Draw the end of a neuron: the foot process of its axon. • Label this the presynaptic cell. • Next, draw the postsynaptic cell, which is the target cell. • Label the space between the two cells the synaptic cleft. • Indicate that the whole site is called the synapse. • Draw a vesicle in the presynaptic cell filled with neurotransmitters, which serve as ligands in this setting. Steps of Chemical Synapsis • Step 1: Show an action potential travel down the presynaptic cell. • Step 2: Show a vesicle fuse with the plasma membrane and release its cargo into the synaptic cleft. - Fusion occurs because the action potential opens voltage-gated calcium channels, and the influx of calcium causes the fusion. • Step 3: Draw a ligand-gated ion channel that it is in the open conformation because the ligand (neurotransmitter) is bound at the binding site. - And show sodium ions (as an example) in the synaptic cleft that traverse the channel to enter the postsynaptic cell due to their electrochemical gradient. - Indicate that there is a slight voltage across the plasma membrane. 3 / 7 • Step 4: Show that when enough ions enter the cell, the membrane depolarizes. - This depolarization can open another type of channel, the voltage-gated ion channel. - Show that the voltage-gated ion channel opens (because of the change in membrane voltage NOT ligand-binding). • Step 5: Show sodium ions pass through the voltage-gated ion channel, which further depolarizes the membrane, opening more voltage-gated ion channels farther along which then allow more ions in. • Step 6: Indicate that this depolarization (the action potential) travels along the membrane as more voltage-gated ion channels open. Connection between cytoplasms of adjacent neurons • Note that in electrical synapses, the cytoplasms of adjacent neurons connect, which allows ions (and action potentials) to travel directly in to the next cell. NEURONAL CODING OF STIMULUS STRENGTH Now let's explore how the nervous system codes for stimulus intensity. • Remember that action potentials are all-or-nothing events, so they are all the same. - That means the nervous system must use some other method to determine intensity – that method is frequency of action potentials. • To understand this concept, first draw a small graph where stimulus strength is the y-axis and time (in ms) is the x- axis. • Draw a dotted-line to indicate the threshold stimulus strength: the stimulus level required to initiate an action potential. • Starting at a stimulus strength of zero, draw three stimuli each increasing in strength. - The first one does not reach the threshold, the second one is slightly stronger than the threshold. - The third one is much stronger than the second stimulus. • Above, draw another graph, labeling the y-axis membrane potential (in mV). • Directly above the first stimulus, draw a straight line at -70mV to indicate that no action potentials are produced because the stimulus did not reach the threshold. • Above the second stimulus, draw 3 action potentials going from -70mV to +30mV. • Finally, above the third stimulus, draw 6 action potentials. - Because the stimulus was greater, there is an increase in the number of action potentials fired in the same time span. How does the stronger stimulus produce more action potentials? - We'll introduce the concept of the refractory period to understand this concept. ABSOLUTE REFRACTORY PERIOD 4 / 7 • Write that the absolute refractory period refers to the time period when the voltage-gated sodium channels are open (during the depolarization phase or immediately afterwards), so there is no way for another stimulus, no matter how strong, to cause produce another action potential. • Thus, despite our stimulus' strength, it can't produce more action potentials during this time period. RELATIVE REFRACTORY PERIOD • But write the relative refractory period refers to the time period when most of the voltage-gated sodium channels have reset and some of the voltage-gated potassium channels are still open. - During this period, it is possible for a stronger than normal stimulus to produce another action potential. • So, indicate that the large stimulus generates more action potentials because of its ability to elicit action potentials during the relative refractory period. MYELIN & NERVE CONDUCTION SPEED See: Myelin & Nerve Conduction Speed Now, let's explore how degree of myelination affects the conduction speed of an action potential. • We will look at three situations: a bare plasma membrane, a non-myelinated axon, and a myelinated axon. Bare plasma membrane • For bare plasma membrane, draw a thin section of cell surrounded by plasma membrane. • Draw two voltage-gated ion channels on the far left side of the membranes. - Indicate that this is where the stimulus starts. • Now draw a voltage arrow that rapidly thins as it travels towards the other end of the section because charged ions leak across the membrane; thus, voltage decreases as it travels until it eventually disappears. Non-myelinated axon • For the non-myelinated axon, draw a section of the axon with multiple voltage-gated ion channels. • Again, apply a stimulus. • Show that it rapidly decreases, but at every voltage-gated ion channel, the voltage is regenerated since new ions are let into the cell to replace the charge that leaks out. - Although the stimulus is regenerated, the action potential conduction speed is slow because it takes time to open new channels to allow ions in. • Draw an arrow to help visualize the action potential traveling along the axon. Myelinated axon 5 / 7 • Finally, for myelinated axons, again draw a section of the axon. • Here, draw the myelin sheath sections along the axon. - Label the nodes of Ranvier between the myelin sheaths and draw voltage-gated ion channels in them.
Details
-
File Typepdf
-
Upload Time-
-
Content LanguagesEnglish
-
Upload UserAnonymous/Not logged-in
-
File Pages7 Page
-
File Size-