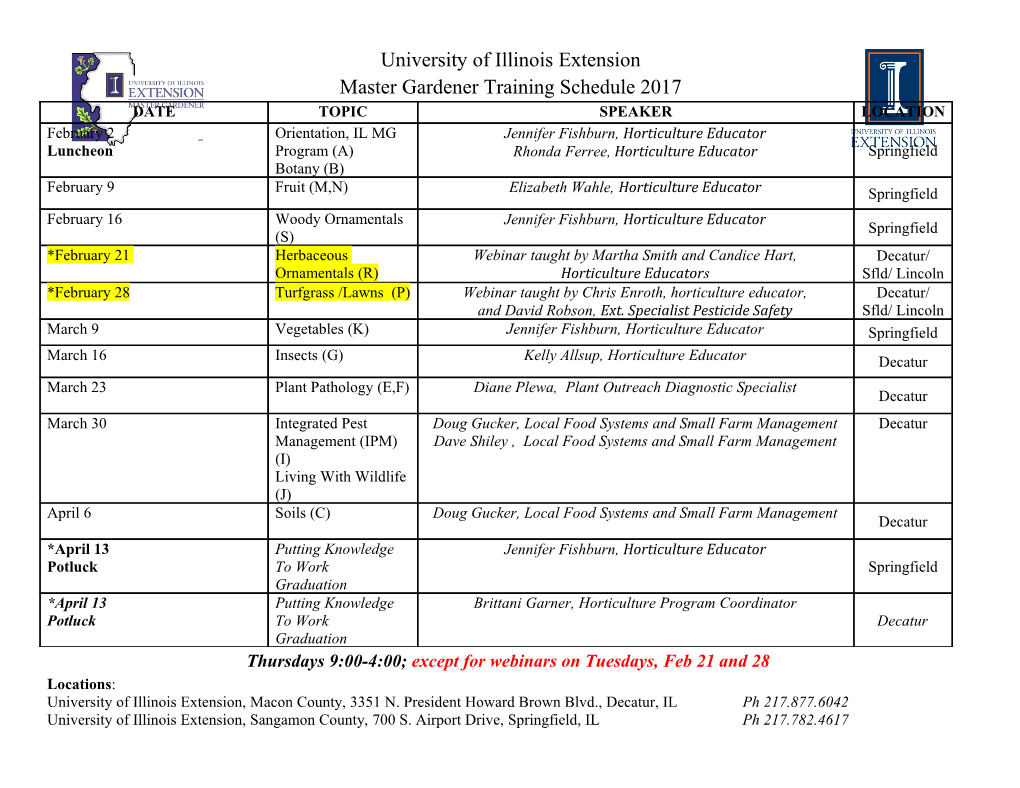
PETROLOGY OF THE 1877 ERUPTION OF COTOPAXI VOLCANO, ECUADOR: INSIGHT ON MAGMA EVOLUTION AND STORAGE Megan Saalfeld A Thesis Submitted to the Graduate College of Bowling Green State University in partial fulfillment of the requirements for the degree of MASTER OF SCIENCE May 2018 Committee: Daniel F. Kelley, Committee Co-Chair Kurt S. Panter, Committee Co-Chair John Farver ii ABSTRACT Daniel F. Kelley, Committee Co-Chair Kurt S. Panter, Committee Co-Chair Cotopaxi is a stratovolcano in the Northern Andes Volcanic Zone, and has a history of bimodal volcanism, alternating between rhyolite and andesite. With Cotopaxi reawakening in 2015 after 138 years of quiescence, the question of what is occurring beneath the surface becomes especially poignant. To contribute to this question, it is productive to look to the volcano's recent past. This work characterizes the mineralogy and geochemistry of the recent eruptive products of Cotopaxi, with emphasis on the two pulses of the 1877 eruption. Additionally, pressures and temperatures are estimated for magmas prior to all eruptions. This will allow a better understanding of the magma plumbing system and its evolution over time. Over the past 500 years Cotopaxi has had five major eruptive events (VEI 3-4), which occurred in 1532, 1742, 1744, 1768, and 1877, and included pyroclastic surges, scoria flows, and lahars. After the initial pulse of the 1877 eruption and the subsequent lahars, a second pulse of magma produced a pyroclastic density current, containing scoria clasts up to 1 meter in diameter. All samples collected from these eruptions are basaltic-andesite to andesite (56-59 wt. % SiO2), with a mineral assemblage of pl + opx + cpx + mag ± ol. Plagioclase from all samples range from An47 to An78 and show both normal and reverse zoning. Normally zoned crystals exhibit greater compositional variation between cores and rims than reversely zoned crystals (median Ancore- Anrim 8% vs 4%, respectively). The presence of disequilibrium textures such as zoned plagioclase and mingled magma indicate that magma mixing plays a role in magma genesis in addition to crystal fractionation. Pyroxenes occur as augite and enstatite and do not exhibit significant iii zoning. The very similar petrologic character of these deposits suggests that they were sourced from a relatively long-lived magma system that underwent differentiation and replenishment between eruptions. Fractional crystallization modeling shows that 17% crystallization of plagioclase (9.8%) + clinopyroxene (3.5%) + olivine (1.6%) + apatite (0.1%) is necessary to produce the observed variation. However, some samples show a better fit with mixing trends than crystallization trends, so it is likely that both processes are occurring. Thermobarometric data indicate that magma storage occurred at temperatures of 1000-1150°C and pressures ranging from 0.20 GPa (during the 1877 eruption) to 0.43 GPa (during the 1532 eruption), which is equivalent to depths of 7 and 15 km (± 9 km), respectively. Data from geodetic and seismic studies indicate that magma was injected at a depth of 4 to 5 km which caused ground deformation and seismic unrest from 2001 to 2002. While thermobarometry is poorly constrained, these results suggest that magma storage has become progressively shallower over time. iv ACKNOWLEDGMENTS I would like to thank the staff of the Instituto Geofisico in Ecuador for collecting and providing samples, and Patty Mothes for inspiring this project. To Craig Grimes from Ohio University for providing assistance with XRF analysis, Tom Darrah at Ohio State University for allowing me access to his lab, Erica Maletic for assistance with ICP-MS, and Gordon Moore at University of Michigan for technical support with EMPA. Microprobe analysis was funded by the Richard D. Hoare Research Scholarship (BGSU). Above all, thank you to my advisors and committee member for teaching me so much over the past two years and for helping me to become a better scientist. v TABLE OF CONTENTS Page CHAPTER 1. INTRODUCTION .......................................................................................... 1 CHAPTER 2. GEOLOGIC SETTING ................................................................................ 3 CHAPTER 3. METHODS AND SAMPLE CHARACTERIZATION ................................ 6 Field Observations ..................................................................................................... 6 Sample Collection ..................................................................................................... 7 Analytical Methods ................................................................................................... 8 CHAPTER 4. RESULTS ...................................................................................................... 10 Petrography ........................................................................................................... 10 Textures Indicative of Magma Mixing .......................................................... 12 Mineral Chemistry ..................................................................................................... 12 Plagioclase ..................................................................................................... 12 Pyroxene ........................................................................................................ 13 Major and Trace Elements ......................................................................................... 13 Glass Compositions ....................................................................................... 13 Whole Rock Compositions ............................................................................ 14 Geochemical Variability Between Eruptions............................................................. 16 Barometry, Thermometry, and Hygrometry .............................................................. 17 Barometry ...................................................................................................... 17 Thermometry.................................................................................................. 18 Hygrometry .................................................................................................... 19 vi CHAPTER 5. DISCUSSION ................................................................................................ 20 Magma Mixing vs. Fractionation ............................................................................... 20 A System of Fractionation and Recharge .................................................................. 24 Magma Storage Conditions........................................................................................ 26 Models of Evolution .................................................................................................. 28 CHAPTER 6. CONCLUSIONS ........................................................................................... 29 REFERENCES.......... ............................................................................................................ 30 APPENDIX A. TABLES ...................................................................................................... 35 APPENDIX B. MICROPROBE ANALYSIS OF PLAGIOCLASE AND PYROXENE COMPOSITIONS................................................................................................................... 48 APPENDIX C. FIGURES .................................................................................................... 55 1 CHAPTER 1. INTRODUCTION Subduction zone volcanism is generated with variable inputs from the partial melting of the subducting slab and sediment, the overlying mantle, and the continental crust (Hickey et al., 1986; Hildreth and Moorbath, 1988; Tatsumi, 1989; Hidalgo et al., 2012). While andesite is one of the most abundant magma compositions occurring at continental volcanic arcs, it has been shown experimentally (Nicholls & Ringwood, 1972; Mysen et al., 1974) and with natural samples (Reubi & Blundy, 2009) that the majority of andesites are not primary melts. Rather, andesitic magmas form as the product of differentiation processes such as crystal fractionation, assimilation and fractional crystallization (AFC) and magma mixing. These processes impart chemical and textural signatures on the resulting lavas (Panter et al., 1997; Schiano et al., 2010; Garrison et al., 2011; Lee and Bachmann, 2014). By determining the role that each of these processes play in the differentiation of magma, we strive to better understand shallow level magma evolution in the context of recent eruptive activity at Cotopaxi. By focusing efforts on a short time interval, one can provide a more detailed account of the processes occurring that have created chemical diversity within the most recent eruptive cycles. Characterizing the storage and evolution of recent magmatism at this classic subduction zone stratovolcano can provide insight into processes occurring here and at similar volcanoes. Cotopaxi is one of the most well-known and well-studied volcanoes in South America. The stratigraphy of its eruptive products has been well documented (Hall and Mothes, 2008; Garrison et al., 2011), and the stratigraphic relationships in the field are very clear, making it easy to distinguish different pulses of eruptions. These factors make Cotopaxi a prime candidate for investigating the magmatic processes that dominate subduction zone volcanism and that cause 2 magmatic variation in the upper crust. This study builds upon previous
Details
-
File Typepdf
-
Upload Time-
-
Content LanguagesEnglish
-
Upload UserAnonymous/Not logged-in
-
File Pages82 Page
-
File Size-