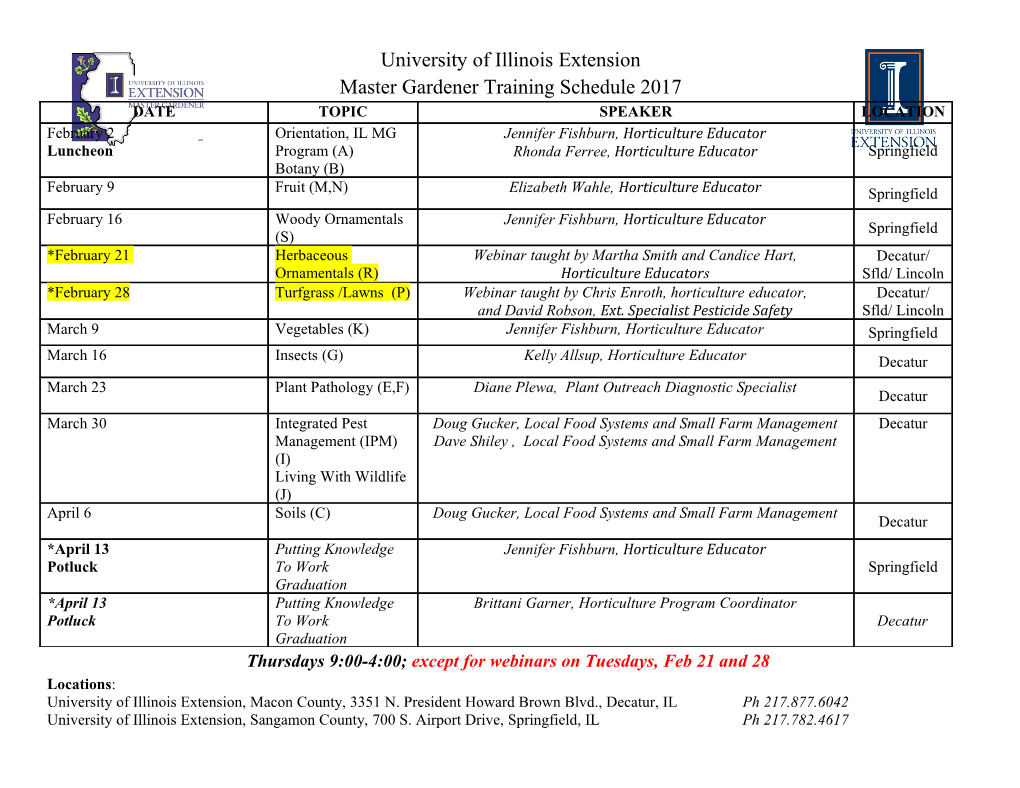
Development and Characterisation of 3D Skeletal Muscle Constructs under Defined Mechanical Regulation By Umber Cheema, B.Sc (Hons) UCL A thesis submitted in partial fulfilment for the Degree of Doctor of Philosophy Royal Free and University College Medical School July 2004 Department of Surgery, Charles Bell House, 64 Riding House Street, London W1W 7EJ. Department of Anatomy and Developmental Biology, Royal Free and University College Medical school, Royal Free Campus, Rowland Hill street, London NW2 2PF 1 UMI Number: U591876 All rights reserved INFORMATION TO ALL USERS The quality of this reproduction is dependent upon the quality of the copy submitted. In the unlikely event that the author did not send a complete manuscript and there are missing pages, these will be noted. Also, if material had to be removed, a note will indicate the deletion. Dissertation Publishing UMI U591876 Published by ProQuest LLC 2013. Copyright in the Dissertation held by the Author. Microform Edition © ProQuest LLC. All rights reserved. This work is protected against unauthorized copying under Title 17, United States Code. ProQuest LLC 789 East Eisenhower Parkway P.O. Box 1346 Ann Arbor, Ml 48106-1346 Acknowledgements I would like to thank my primary supervisor, Robert Brown, for all his help, encouragement, guidance and support over the course of my studies. He has helped make this whole learning experience a truly enjoyable one. I am also deeply thankful for the support of my second supervisor, Geoffrey Goldspink, who has given me a sound understanding of muscle and for giving me the opportunity to study. I would like to thank all the staff and students I have had the privilege of working with. I cannot name them all, but without their constant support and words of encouragement I certainly wouldn’t be in my current position. I would like to thank especially some of my colleagues; Massimo, Zoe, Rebecca, James, Sally, Oksana, Dimitris, Neelam, Mahjabeen, Vivian, Kirsty, Vivek and Shi- Yu. I realise how lucky I have been to have stumbled across such brilliant, charismatic and intelligent beings, and I wish them all the best. I would also like to thank my best friend for giving me someone to admire. My family, both near and far, have been there throughout all my frustrations. Without their encouragement and reassurance I couldn’t have coped. I would especially like to thank my parents for everything they have given me, this thesis is for them. 2 Abstract It has been shown that the IGF-I gene is spliced in response to mechanical signals producing forms of IGF-I which have different actions. To study the roles of environmental mechanical cues on gene programming and splicing in developing muscle, an in vitro 3D cell culture system was employed. C 2C 12 skeletal myoblasts were grown in 3D collagen cultures. The presence of this extracellular matrix component, the application of uniaxial strain (produced endogenously by the myoblasts) and a high concentration of cells was shown to enhance differentiation in these cultures. These differentiated myotube cultures were then subjected to different regimens of exogenous mechanical strain. IGF-IEa, which initiates the fusion of myoblasts to form myotubes, was found to be constitutively expressed in myoblasts and myotubes and its expression up-regulated by a single ramp stretch of one hour duration but reduced by repeated cyclical stretch. In contrast, MGF which is involved in the proliferation of mononucleated myoblasts, that are required for secondary myotube formation and to establish the muscle satellite (stem) cell pool, showed no significant constitutive expression in static cultures, but was up-regulated by a single ramp stretch and by cycling loading. The latter types of force simulate those generated in myoblasts by the first contractions of myotubes. These data indicate the importance of understanding the physiological signals that determine the ratios of splice variants of some growth factor/tissue factor genes in the early stages of development of skeletal muscle. 3 Contents Page Title Page 1 Acknowledgements 2 Abstract 3 Contents Section 4 - 5 List of Figures 6-8 Introduction 9- 64 Overview 9 Skeletal Muscle 13 Dystrophin 30 3D tissue engineering 32 Development o f tissue engineered skeletal muscle cultures 37 Mechano-transduction across the cell 42 Insulin-like growth factors (IGFs) 49 Hypothesis 63 Aims o f study 63 Materials and Methods 65-106 Basic Methods 65 Real time Quantitative PCR 97 Results 107-163 Results section 1: Collagen Material Properties 107 4 Results section 2: Development of 3D skeletal model 115 Results section 3: Morphological characterisation of 133 differentiating 2CC 12 myoblasts in 3D Results section 4: Skeletal myotube model responses to 147 applied mechanical loads Discussion 163-209 Original hypothesis 163 Conclusions 164 Future work 208 References 210- 247 Publications 248 5 List of figures Introduction Page Figure 1.1 A representative diagram of the structure of skeletal muscle 14 Figure 1.2 Diagrammatic representation of a sarcomere 17 Figure 1.3 A diagrammatic representation of the processes involved 24 in the repair of a skeletal muscle fibre following damage Figure 1.4 A diagrammatic representation of the cell cycle 29 Figure 1.5 Diagrammatic representation of cell matrix remodelling 34 Figure 1.6 A diagrammatic representation of pro-insulin 51 Figure 1.7 Schematic of the IGF-IR 54 Figure 1.8 A schematic representation of the IGF-I gene 60 Materials and Methods Figure 2.1 The three tissue layers of skin 66 Figure 2. 2 Collagen gel set-up 69 Figure 2.3 A schematic diagram showing the contraction of the 70 myoblast/myotube collagen culture Figure 2.4 A schematic diagram representing the set-up of the CFM 71 Figure 2.5 The CFM instrument setup 72 Figure 2.6 This picture shows the t-CFM set-up 73 Figure 2.7 A calibration curve for a force transducer 74 Figure 2.8 Patterns of cyclical load applied to myotube tissue cultures 79 over one hour Figure 2.9 The patterns ramp loading regimens applied to myotube 81 tissue cultures Figure 2.10 Pictures showing different myoblast density plated over 85 varying time periods and the differentiation of these cells Table 2.1 Description of experimental protocols used for PCR 89 amplification of both IGF-IEa and MGF Table 2.2 Primers used for cDNA synthesis step and PCR step given 90 in the 5’ to 3’ direction Figure 2.11 Picture of the lightcycler rotor 91 Figure 2.12. Picture of dsDNA 98 Figure 2.13. The melting curve analysis for GAPDH 101 Figure 2.14. This graph highlights the cycle number at which the target 102 starts amplifying in the logarithmic phase Figure 2.15. A plot of the standards points, with a line of best fit 103 Figure 2.16. Real Time quantitative data screen 104 Figure 2.17. Melting curve analysis for MgCl run with IGF-IEa primers 105 Figure 2.18 DNA products run on an agarose gel 106 Results Figure 3.1. Graph showing 10% strain applied to the collagen gel, 109 over a 10 minute period Figure 3.2. Deformation of the collagen gel lengthways results in three 109 changes of the collagen gel Figure 3.3. 10% strain was applied to the culture over 1 hour 110 (10% strain per hour) Table 3.1. A table to show the total amount of strain retained by the 111 collagen matrix Figure 3.4. Diagrammatic representation of one cycle of loading on the 112 collagen gel, using the t-CFM Figure 3.5. Graph showing 1 cycle per hour loading regimen applied to a 113 plain collagen gel Figure 3.6. Graph showing 5 cycle per hour loading regimen applied to 114 a plain collagen Table 3.2. A table showing increases in the total amount force required to 114 deform the matrix by 1% Figure 3.7. Comparison of typical contraction profiles from three cell types 116 Figure 3.8. Comparison of mean rates of contraction for three cell types 117 Figure 3.9. Three day mean force profile for myoblasts 119 Figure 3.10. Histogram showing the mean gradient for force generation 120 (rate of change of force) over phases 1 to 5 Figure 3.11. Morphology of myoblasts in 3D collagen matrix 121 Figure 3.12. A collagen gel after it has been set in a rectangular mould and 123 24 hours after cell contraction Figure 3.13. Alignment of myoblasts within a CFM collagen gel 124 Figure 3.14. Myoblasts embedded within a collagen gel on day three 125 visualised using light microscopy Figure 3.15. Morphology of myoblasts in different regions of the collagen gel 127 Figure 3.16. Levels of IGF-IEa and MGF mRNA in differentiating myoblasts 129 in a 3D collagen matrix Figure 3.17. Phalloidin staining of myotubes in a tethered collagen gel 130 Figure 3.18. A typical myotube contraction curve 131 Table 3.3. Statistical analysis showed the time points at which the jumps 132 occurred Figure 3.19. Myoblasts on day 3 after being seeded into the collagen gel 134 Figure 3.20. Unidirectional alignment of myoblasts is shown 136 Figure 3.21. Light micrograph of myoblasts on day 3 138 Figure 3.22. Low magnification electron micrograph to show two nuclei in 140 a myotube with complete membrane degradation between the fused cells in the fusion zone Figure 3.23. Alignment of both myotubes and collagen fibrils (CF) within the 142 7 10 day culture Figure 3.24. The elongated, aligned myotubes within the collagen gels 143 exhibited banding in their nuclei Figure 3.25. Alignment of myotube in day 10 gel along the line of principal 145 strain (double-headed arrow), with banding of nuclei and a large amount of golgi apparatus Figure 3.26.
Details
-
File Typepdf
-
Upload Time-
-
Content LanguagesEnglish
-
Upload UserAnonymous/Not logged-in
-
File Pages248 Page
-
File Size-