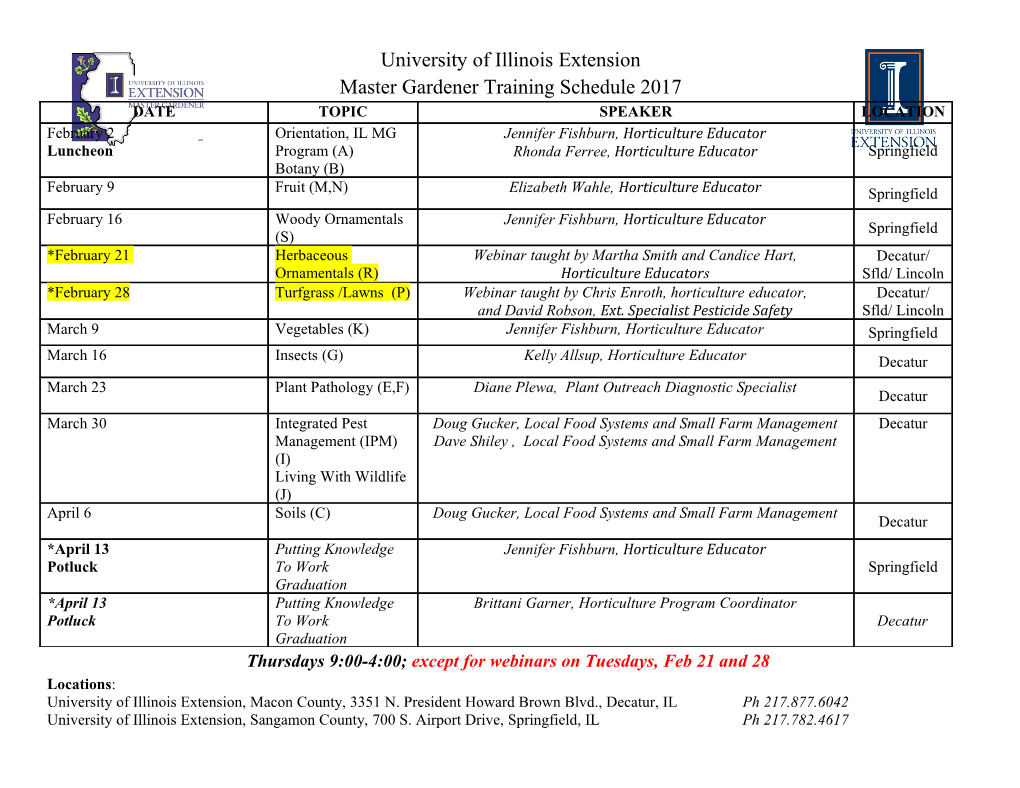
J.E. MENARD et al. FUSION ENERGY DEVELOPMENT APPLICATIONS UTILIZING THE SPHERICAL TOKAMAK AND ASSOCIATED RESEARCH NEEDS AND TOOLS J.E. MENARD, R. MAJESKI, M. ONO Princeton Plasma Physics Laboratory Princeton, NJ USA Email: [email protected] N.N. BAKHAREV, V.K. GUSEV Ioffe Institute Saint Petersburg, Russia M. GRYAZNEVICH, D. KINGHAM, S. MCNAMARA, P. THOMAS Tokamak Energy Ltd Oxfordshire, United Kingdom K. HANADA Kyushu University Fukuoka, Japan J. HARRISON, B. LLOYD Culham Centre for Fusion Energy (CCFE) Oxfordshire, United Kingdom Y.S. HWANG Seoul National University Seoul, South Korea B. LIPSCHULTZ, H. WILSON (also CCFE) University of York York, United Kingdom Y. NAGAYAMA Nihon University Tokyo, Japan Y. ONO, Y. TAKASE The University of Tokyo Tokyo, Japan M. REINKE Oak Ridge National Laboratory Oak Ridge, TN, USA K. TOBITA National Institutes for Quantum and Radiological Science and Technology Aomori, Japan Z. GAO Tsinghua University Beijing, China F. ALLADIO ENEA Rome, Italy R.J. FONCK University of Wisconsin-Madison Madison, WI, USA 1 IAEA-CN-258 OV/P-6 Abstract The spherical torus/tokamak (ST) is a potentially attractive configuration for narrowing scientific and technical gaps to a fusion demonstration power plant and as a more compact and/or modular fusion power source. Due to a reduced plasma surface area to volume ratio, the ST configuration offers the potential to access high power exhaust heat fluxes and high neutron wall loading in devices of modest size. Further, due to increased ability to utilize magnetic pressure to confine a given plasma pressure, the ST may also offer fusion solutions with reduced magnet mass and increased mass power density. Potential fusion development and energy production applications of the ST include: divertor and first-wall heat-flux mitigation research and development (including tests of advanced divertor configurations and liquid metals), neutron sources with low-to-moderate fusion gain (Q=0.1-1) for hybrid applications, neutron sources with moderate gain (Q=1-5) for fusion nuclear science and component testing including breeding blanket development, higher gain (Q=5-10) systems for electricity break-even in a pilot plant, and high gain (Q > 10) modular (Pelec = 100-500MWe) and larger-scale (Pelec = 500-1000MWe) fusion power plants. Details of these applications including major scientific and technology needs and gaps for fusion energy development are described in this paper. Motivations for utilizing the ST for these applications and remaining physics and technological gaps to be addressed to realize the application are also discussed. 1. INTRODUCTION The spectrum of scientific and technological gaps that must be closed to achieve practical fusion energy has been extensively documented in detailed reports both within the U.S. [1] and internationally [2,3,4,5]. A common barrier to narrowing or closing these gaps is the scale and cost of fusion facilities needed to address these gaps. For tokamaks, this motivates consideration of alternatives to the conventional aspect ratio tokamak. The low-aspect-ratio spherical torus/tokamak (ST) is a potentially attractive configuration for narrowing scientific and technical gaps to a fusion demonstration power plant and as a more compact and/or modular fusion power source in its own right. A distinguishing feature of the ST is a higher ratio of plasma pressure to externally applied magnetic field pressure and the corresponding potential for reduced mass and cost of the toroidal field magnets and fusion core in general. Due to the reduced plasma surface area to volume ratio, the ST configuration offers the potential to access high power exhaust heat fluxes and high neutron wall loading in devices of modest size. Potential challenges for the ST approach include the necessity to generate/ramp-up and sustain the plasma current non-inductively. Further, the physics basis – in particular, for energy confinement – is not as well established as it is in conventional tokamaks. However, several upcoming experiments aim to significantly expand the ST physics basis and provide more confidence in projecting the performance of next- step ST facilities. In the sections that follow, details of a range of ST applications are described, including major scientific and technology needs and gaps for fusion energy development. Further, motivations for utilizing the ST configuration for these applications and remaining physics and technological gaps to be addressed to realize the application are also discussed. 2. FUSION DEVELOPMENT APPLICATIONS OF THE ST Fusion development paths and device applications can be organized according to both performance gaps and projected fusion performance / fusion gain. In this paper, ST applications Sections are organized as follows: 2.1 Developing solutions for the plasma-material-interface (PMI) challenge 2.2 Fusion-fission hybrid systems 2.3 Developing fusion components capable of withstanding high fusion neutron flux and fluence 2.4 Demonstrating electricity break-even from a pure fusion system 2.5 Electricity production in modular fusion power plants 2.6 Electricity production in larger-scale fusion power plants NOTE: The work described here is a survey of potential ST applications and inclusion of a particular application. This does not necessarily represent endorsement of that application by all co-authors. 2.1. Developing solutions for the plasma-material-interface (PMI) challenge In order for the ST to support the discussed fusion development applications, a set of key experimental milestones need to be achieved in the area of boundary science, primarily using NSTX-U [6,7], MAST-U [8,9,10], and ST40 over the next decade. These provide the existence proofs that are necessary but not sufficient for future operational scenarios They will provide both important information to scope new engineering designs using empirical models and generate data to support validation of modeling and simulation tools. Unordered for priority they are: J.E. MENARD et al. 2.1.1. Develop a Consistent Empirical Heat Flux Width Scaling Across STs Predicting the parallel heat flux, 푞∥ = 푃푆푂퐿퐵/2휋푅휆푞퐵푝 , enables designs of plasma facing components (PFCs) and sets boundary conditions for heat flux mitigation scenarios. Conventional aspect ratio devices have given consistent results when overlapping in engineering parameters, and recently Alcator C-Mod has confirmed the narrowing of the heat flux width out to Bp ~ 1.3 T [11]. For STs, there are presently multiple predictions which 0.42 0.04 −0.92 −0.02 differ from the multi-machine scaling: 휆푞,푀푀[푚푚] = 1.35휀 푅푔푒표 퐵푝표푙,표푚푝푃푆푂퐿 (Table 3, Regression #15 in Reference [12]). MAST found a much weaker poloidal field dependence and a stronger dependence on power into the scrape-off layer for H-modes, (Table 2 and Regression #2 in Reference [13]): 휆푞,푀퐴푆푇[푚푚] = −0.68(±0.14) 0.18(±0.07) 1.84(±0.48)퐵푝표푙,표푚푝 푃푆푂퐿 . In contrast, NSTX as well as Globus-M predicted a stronger variation −1.6±0.1 with plasma current than is seen on conventional aspect ratio devices: 휆푞,푁푆푇푋[푚푚] = 9.1퐼푝 [14] and -1.4+/-0.2 λq,Globus-M [mm] ~ Ip [15]. Moreover, a further narrowing during operation with lithium evaporation was observed NSTX. When estimated for cases for the full operating parameters of NSTX-U the scalings give 휆푞,푀푀 = 2.0 푚푚, 휆푞,푀퐴푆푇 = 4.1 푚푚, 휆푞,푁푆푇푋 = 3.0 푚푚. Such a disagreement, achievable within both MAST- U and NSTX-U operating space, will be observable within resolution of diagnostic sets, and experiments to explore heat flux width scalings are identified as high priority areas for early device operations. The ST40 tokamak, designed to operate up to Bp ~ 1T, will also make critical contributions in confirming how strong the narrowing is of 휆푞 with poloidal field, reducing the step between near-term machines and those listed in Table 1 supporting fusion energy development. 2.1.2. Combine High Performance Pedestals with Exhaust Solutions at High Parallel Heat Flux in the ST The present class of STs are currently right on the border of necessitating heat flux mitigation to support operation. For double null, NSTX-U 2 MA, 1 T, 10 MW PNBI scenarios, PFCs are being designed sustain 5 second flattops [6] using a combination of poloidal flux expansion and strike-point sweeping, assuming onlyonly 30% radiated power. ST40, while at high heat flux, is short pulse allowing for inertially cooled PFCs and MAST-U’s large divertor surface area and flexible divertor PF coil set would allow, in absence of radiation, a large surface area for sweeping. Facilities used in fusion development applications will increase in power density and pulse length, necessitating increased radiated power in the core and boundary and active cooling, assuming a paradigm of solid material PFCs. Thus what is a presently a physics research topic becomes an existential operational issue for the next step. Strategies to address this could include using the early commissioning phase where the learning is done in-situ (much like planned ITER low-field operations) or an interim facility to demonstrate core-edge integration in the ST. Fortunately, the new generation of STs will be able to investigate all conventional and advanced divertor scenarios via the combined and coordinated exploitation of NSTX-U and MAST-U. MAST-U will provide the fusion community with the first demonstration of a baffled and pumped divertor with high toroidal flux expansion (e.g the Super-X divertor). In contrast the baseline design of NSTX-U will utilize an open divertor and flat horizontal (vertical) plates for its outer (inner) divertor, but be able to operate at higher power and pedestal pressures. Both have sufficient inner poloidal field coils to support high poloidal flux expansion (e.g. X-divertor) and higher order poloidal field nulls (e.g. Snowflake divertors). Parallel heat fluxes are expected to reach 100’s of MW/m2, where volumetric losses by impurities are necessary.
Details
-
File Typepdf
-
Upload Time-
-
Content LanguagesEnglish
-
Upload UserAnonymous/Not logged-in
-
File Pages12 Page
-
File Size-