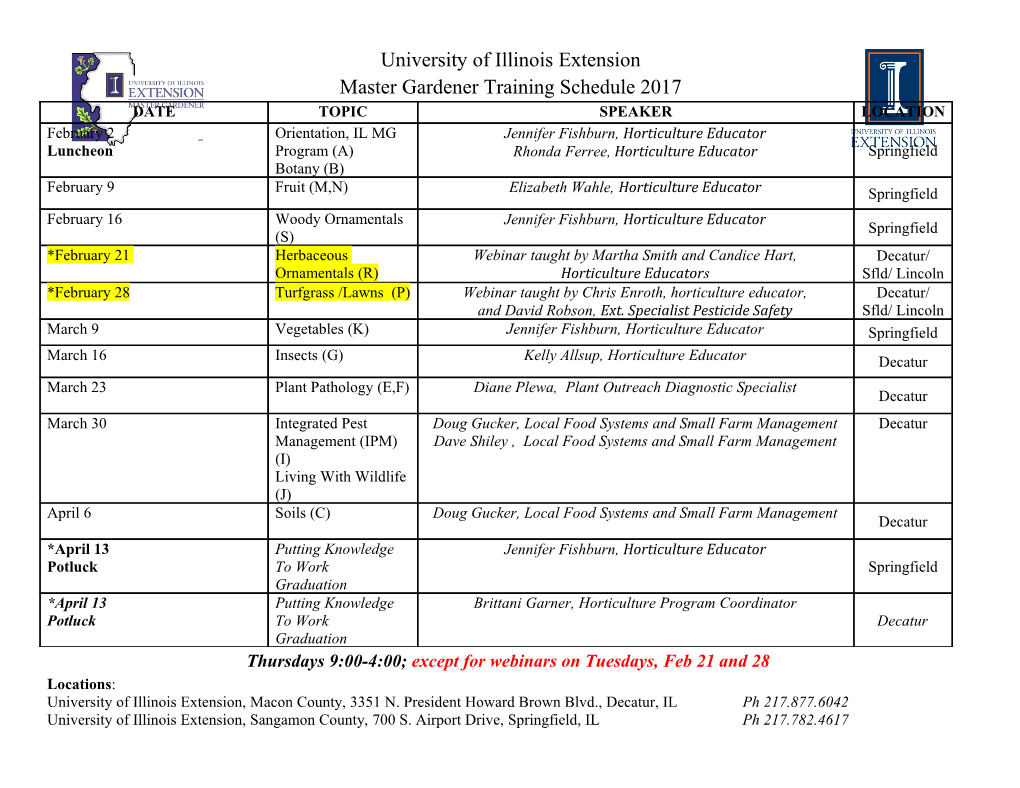
Nuclear Magnetic Resonance Analysis of Flavonoids Tom J. Mabry, Jacques Kagan, Heinz Rosler HO OH 0 THE UNIVERSITY OF TEXAS PUBLICATION AUSTIN, TEXAS Nuclear Magnetic Resonance Analysis of Flavonoids Tom J. Mabry, Jacques Kagan, Heinz Rosler Department of Botany and Cell Research Institute The University of Texas, Austin, Texas THE UNIVERSITY OF TEXAS PUBLICATION NUMBER 64I8 SEPTEMBER I5, Ig64 PUBLISHED TWICE A MONTH BY THE UNIVERSITY OF TEXAS, UNIVERSITY STATION, AUSTIN, TEXAS, 78712. SECOND-CLASS POSTAGE PAID AT AUSTIN, TEXAS. Contents PAGE Acknowledgments 4 Introduction . 5 Materials and Methods 6 Interpretation of NMR Spectra of Trimethylsilyl Ethers of Flavonoids 7 Discussion 10 Literature Cited 10 NMR Spectra 1-51 12 Acknowledgments This investigation was supported by Grant F-130 from the Robert A. Welch Foundation, The National Institutes of Health Grant GM"l 1111-02 and the sup· plemental grant NIH-GM-ll l 1 l-02S1. One of u~, J. K, thanks the Robert A. Welch Foundation for a Post-doctoral Fellowship, 1963-1965. The authots thank the Chemistry Departments of Rice University, The University of Texas and Texas Christian University for the use of Varian A-60 spectrometers. Many of the flavonoids used in this investigation wern generously provided by Margaret Seikel, J. Herran, A. R. Kidwai1 H. Wagrtet, E. W. Underhill, E. M. Bickoff, R. Neu, F. De Eds, M. Hasegawa, Artnn Nilsson and J. Chopin. The editorial assistance of Ursula Rosler, Myra Mabry and G. Knipfet is grate­ fully acknowledged. Finally, we thank the Graduate School of The Utiiversity of Texas for grant SRF-289 for publication support. Nuclear Magnetic Resonance Analysis of Flavonoids ToM J. MABRY, jACQUEs KAGAN, and HEINZ RosLER* Department of Botany and Cell Research Institute The University of Texas, Austin Introduction Flavonoids constitute a large class of secondary compounds, widespread in the higher plants, which are especially useful for taxonomic purposes at the species level. In an extensive biochemical systematic investigation of the genus Baptisia (family Leguminosae), flavonoid patterns, as disclosed by two-dimensional paper chromatography, were used to validate natural hybridization and to study the structure of populations.1 Subsequently, this work was extended to include not only the isolation and chemical analysis of the Baptisia flavonoids but also those from selected genera of other families. Although the isolation of natural products frequently requires elaborate pro­ cedures, the tools of modern organic chemical analysis, gas chromatography and nuclear magnetic resonance ( NMR), infra-red, visible-ultraviolet, and mass spec­ troscopy, often allow rapid structure analysis of pure substances without time­ consuming chemical degradations and syntheses. One of the more recent tech­ niques, NMR spectroscopy, has had limited application for naturally occurring flavonoids, most of which are glycosides, because of their low solubility in most 2 organic solvents. ·• Common derivatives, such as methoxy and acetyl, are generally not suitable for the NMR analysis of all flavonoids because the signal pattern of the natural product is often obscured, in part, by the signals of the additional groups. Following the report of Sweeley and co-workers5 for preparing the trimethylsilyl ethers of carbohydrates, we investigated the potential of these derivatives for the NMR analysis of flavonoids. 2 Independently, and using a different procedure Waiss, Lundin and Stern3 reported NMR data for the trimethylsilyl ethers of several flavonoid aglycones. Subsequently, Batterham and Highet•b described an alternate method in which they used deuterated dimethylsulfoxide. Most flavonoids are soluble in this solvent and the identification of a large number of them was reported. In our work, we have analyzed, in particular, the NMR spectral patterns displayed by the sugar components of flavonoid glycosides. We have found the readily prepared carbon tetrachloride-soluble trimethylsilyl ethers satisfactory for the NMR analysis of all flavonoids thus far examined. We believe the availability of the actual NMR spectra is of considerable value for structural studies since most tabular presentations of NMR data do not provide adequate descriptions of the spectra in all detail. *Permanent address of H.R.: Institut fiir Pharmazeutische Arzneimittellehre der Universi­ tat Miinchen. 6 Nuclear Magnetic Resonance Analysis of Flavonoids Materials and Methods The following conditions were typically employed for preparing the trimethyl­ silyl ether derivatives of flavonoids (Figure 1). Fifty mg of substance was dissolved BcH,),sD2"H (Cff,)3SiCI pyndlne OH~ OS HCH,>J rhafHOfilCCIH-0 lllulri:i.=::z:· \ llCll, ri 1-0 OCH 3 ~ OH aqu .lleOlt HESPERIDIN SILYLATED HESPERIDIN Figure 1. Trimethylsilylation and hydrolysis procedure. in 3 ml of pyridine and treated successively with about 0.5 ml of hexamethyldisila­ zane and 0.5 ml of trimethylchlorosilane (Applied Sciences Products, State Col­ lege, Pa.) . The solvent and excess reagents were immediately removed under high vacuum and the dry residue was extracted with carbon tetrachloride. The clear carbon tetrachloride solution obtained by filtering off the salts was ready for NMR analysis when concentrated to a suitable volume. These steps require about 20 minutes. The original substance could often be regenerated unaltered by allowing the trimethylsilyl ether to stand several hours or to reflux 30 minutes in 50 ml of 20% aqueous methanol usually with a drop of acetic acid. Frequently the flavo­ noid crystallized directly, otherwise the hydrolyzed product was chromatographed over silica gel or polyamide. The same procedure was used for all phenolics and sugars investigated. However, it was observed that the total conversion of flavo­ noids with sterically hindered hydroxyl groups to their trimethylsilyl ether often required a reaction time of more than one hour at room temperature. We subse­ quently observed that the addition of a small amount of trimethylsilyl chloride and hexamethyldisilizane into the NMR tube prevented hydrolysis and insured com­ plete trimethylsilylation. The NMR spectra were obtained on a Varian A-60 spec­ trometer in carbon tetrachloride. In most instances, tetramethylsilane (TMS) was an internal standard, but similar results were observed when external TMS in carbon tetrachloride was used as reference. Mabry, Kagan and Rosier 7 Interpretation of NMR Spectra of Trimethylsilyl Ethers of Flavonoids All hydroxyl groups in both the aglycones and glycosides were converted to their trimethylsilyl ethers as shown by the integration of the NMR spectra. In contrast to methoxy and acetyl derivatives of flavonoids, the NMR signals arising from the trimethylsilyl groups occur out of the absorption region of protons in flavonoids, at about 2.0 ppm from tetramethylsilane. Most of the signals from the silyl groups occur downfield from TMS, however groups attached at C-3 in some flavonoids are found at about 0.1 ppm upfield with respect to TMS (spectra 32- 35). Occa­ sionally the trimethylsilyl group at the 5-position was hydrolyzed when the deriva­ tive was exposed to a moist atmosphere. The C-5 hydroxyl group was then readily detectable since the proton, which is hydrogen-bonded to the C-4 keto group, gives a singlet near 13 ppm (cf. spectrum 2). The hydroxyl substitution pattern of the flavonoid nucleus as well as the position of glycosidation and the nature of the sugar moiety in glycosides can be deduced from the spectra of these trimethyl­ silylated derivatives. 8 0 1 c 2 4' 6 3 0 Figure 2. Numbering system for flavonoids. A-ring Protons The two A-ring protons of flavonoids with the usual 5,7-hydroxylation pattern give rise to two doublets (J meta = 2 .5 cps) between 6.0- 6. 7 ppm from tetramethyl­ silane. There are however, small but predictable variations in the chemical shifts of the C-6 and C-8 proton signals depending on the 5- and 7- substituents. In the spectra of the four luteolin derivatives ( 1-4) which vary only in the C-5 and C-7 substituents, the B-ring protons display practically identical signal patterns. In con­ trast, in the spectrum of 7 ,3', 4' -tri-trimethylsiloxy-luteolin ( 2), the C-3 proton signal is shifted downfield while the C-8 proton is shifted upfield, each about 10 cps from their positions in the spectrum of the totally trimethylsilylated luteolin ( 1). 8 Nuclear Magnetic Resonance Analysis of Flavonoids The signal of the C-6 proton ( 6.18 ppm) does not depend on the presence of the trimethylsilyl group at C-5 (cf. spectra 1, 2, 3, and 4). When glycosidation occurs in the A-ring ( c.f. spectra 3, 4, 6, 12, 16, 18, 39, 41, 43, and 45), the patterns for the signals of the A-ring protons differ slightly with respect to the aglycones, while the B- and C-ring proton signals for these glycosides compare closely with the corresponding signals in their aglycones. The NMR pat­ terns of the A-ring protons of ftavonoids that have sugars attached at either C-3 or C-4' (spectra 22, 23, 24, 29, and 31) are usually similar to those observed for their respective aglycones. If a hydrogen has replaced a hydroxyl group at C-5, the C-4 keto group dia­ magnetic-anisotropically deshields this C-5 proton which appears near 8.0 ppm (spectra 26, 35, and 36 ). Flavonoids frequently have substituents at C-6 or C-8 and their assignment by conventional methods is often difficult. Furthermore, the well known Wessely-Moser reaction tends, in effect, to equilibrate a substituent between these two positions by opening of the ring C and closure in the alternate position. However, the NMR signals for the C-8 proton generally occur downfield with respect to the C-6 proton, thus providing a method for ascertaining the position of the substituent. This technique allowed Hand and Horowitz6 to propose structures for the isomeric carbon glycosides vitexin and saponaretin ( c.f. spectra 9 and 10). 8-ring Protons All B-ring protons absorb around 6.7-7.7 ppm, a region separate from the usual A-ring protons.
Details
-
File Typepdf
-
Upload Time-
-
Content LanguagesEnglish
-
Upload UserAnonymous/Not logged-in
-
File Pages44 Page
-
File Size-