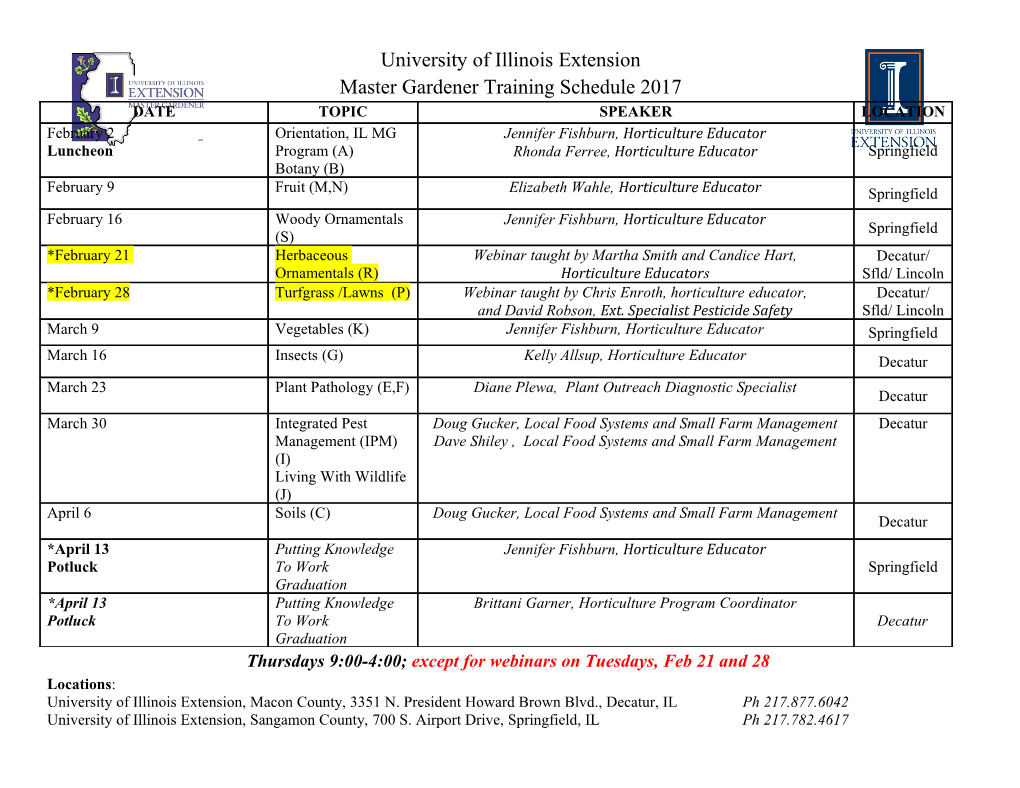
Redox chemistry changes in the Panthalassic Ocean linked to the end-Permian mass extinction and delayed Early Triassic biotic recovery Guijie Zhanga, Xiaolin Zhanga, Dongping Hua, Dandan Lia, Thomas J. Algeob, James Farquharc, Charles M. Hendersond, Liping Qina, Megan Shene, Danielle Shene, Shane D. Schoepferd, Kefan Chena, and Yanan Shena,1 aSchool of Earth and Space Sciences, University of Science and Technology of China, Hefei 230026, China; bDepartment of Geology, University of Cincinnati, Cincinnati, OH 45221; cDepartment of Geology & Earth System Science Interdisciplinary Center, University of Maryland, College Park, MD 20742; dDepartment of Geoscience, University of Calgary, Calgary, AB, Canada T2N 1N4; and eThomas S. Wootton High School, Rockville, MD 20850 Edited by Mark H. Thiemens, University of California at San Diego, La Jolla, CA, and approved December 23, 2016 (received for review July 5, 2016) The end-Permian mass extinction represents the most severe biotic (50.67542°N, 115.07992°W), which represents an outer shelf or crisis for the last 540 million years, and the marine ecosystem upper slope setting in the eastern Panthalassic Ocean at a paleo- recovery from this extinction was protracted, spanning the latitude of ∼30°N (21, 22) (Fig. 1). A second set of study samples is entirety of the Early Triassic and possibly longer. Numerous studies from Gujo-Hachiman in central Japan (35.7355°N, 136.8489°E), from the low-latitude Paleotethys and high-latitude Boreal oceans which represents the abyssal plain of the equatorial Panthalassic have examined the possible link between ocean chemistry changes Ocean (23) (Fig. 1). and the end-Permian mass extinction. However, redox chemistry The Opal Creek section consists of black shale and siltstone of changes in the Panthalassic Ocean, comprising ∼85–90% of the the lower Phroso Siltstone Member and upper Vega Siltstone global ocean area, remain under debate. Here, we report multiple Member of the Sulfur Mountain Formation (Fig. 2). The lowermost S-isotopic data of pyrite from Upper Permian–Lower Triassic deep- Sulfur Mountain Formation contains abundant siliceous monaxon sea sediments of the Panthalassic Ocean, now present in outcrops of sponge spicules and triaxon hyalosponge spicules that disappear western Canada and Japan. We find a sulfur isotope signal of neg- abruptly at 0.36 m above the base of the formation (20). The dis- Δ33 δ34 δ34 ative S with either positive Sornegative Sthatimplies appearance of sponge spicules coincided with a sudden influx of δ34 mixing of sulfide sulfur with different S before, during, and after Late Permian conodonts belonging to the genus Clarkina,marking the end-Permian mass extinction. The precise coincidence of the the onset of the end-Permian extinction event (20) (Fig. 2). Four Δ33 negative S anomaly with the extinction horizon in western Can- conodont zones were defined at Opal Creek, with the first occur- ada suggests that shoaling of H2S-rich waters may have driven the rence of Hindeodus parvus and Clarkina taylorae indicating the end-Permian mass extinction. Our data also imply episodic euxinia Permian–Triassic boundary (20, 24) (Fig. 2). and oscillations between sulfidic and oxic conditions during The Gujo-Hachiman section consists of three units: Unit I, the earliest Triassic, providing evidence of a causal link between comprising green–gray ribbon-chert; Unit II, comprising green– incursion of sulfidic waters and the delayed recovery of the gray to black siliceous claystone; and Unit III, comprising black marine ecosystem. shale with a few thin lenses of gray chert and layers of white– yellow siliceous claystone bearing uppermost Permian radiolarians end-Permian mass extinction | Panthalassic Ocean | multiple sulfur (17, 18, 25) (Fig. 2). Radiolarian and conodont data suggest that isotopes | sulfidic waters Significance he end-Permian mass extinction was the largest biotic ca- Ttastrophe of the last 540 million years, resulting in the dis- appearance of >80% of marine species, and a full biotic recovery To understand how most life on Earth went extinct 250 million did not occur until 4–8 million years after the extinction event years ago, we used multiple sulfur isotopes to investigate re- – dox chemistry changes in the Panthalassic Ocean, comprising (1 6). Several lines of evidence from the low paleolatitude ∼ – Paleotethys and high paleolatitude Boreal oceans, which accounted 85 90% of the contemporaneous global ocean. The S-isotopic for ∼10–15% of the contemporaneous global ocean area, suggest anomalies from Canada and Japan provide evidence for the timing of the onset of euxinia and mixing of sulfidic and oxic that sulfidic (H2S-rich) conditions may have developed widely during the end-Permian extinction (7–13). However, redox chem- waters. Our data suggest that shoaling of H2S-rich waters may istry changes in the Panthalassic Ocean, comprising ∼85–90% of the have driven the mass extinction and delayed the recovery of global ocean area, remain controversial, with competing hypotheses the marine ecosystem. This study illustrates how environmen- tal changes could have had a devastating effect on Earth’s proposing extensive deepwater anoxia (“superanoxic ocean”)or early biosphere, and may have present-day relevance because suboxic deep waters in combination with spatially constrained global warming and eutrophication are causing development thermoclineanoxia(14–18). Evidently, redox chemistry changes in of sulfidic zones on modern continental shelves, threatening the Panthalassic Ocean are central to an examination of the links indigenous marine life. between global-ocean conditions and the end-Permian extinction event as well as the subsequent delayed biotic recovery. – Author contributions: Y.S. designed research; T.J.A., C.M.H., and Y.S. collected samples; The preservation of Permian Triassic boundary deep-sea G.Z., X.Z., D.H., D.L., J.F., L.Q., M.S., D.S., K.C., and Y.S. performed geochemical analysis; sediments is limited because most oceanic crust of that age has G.Z., X.Z., D.H., D.L., T.J.A., J.F., C.M.H., L.Q., S.D.S., K.C., and Y.S. analyzed data; and G.Z. been subducted, and the only surviving Panthalassic seafloor and Y.S. wrote the paper. sediments are within accretionary terranes or marginal uplifts The authors declare no conflict of interest. now located in western Canada, Japan, and New Zealand (19, This article is a PNAS Direct Submission. 20). In this study, we report analyses of all four sulfur isotopes Freely available online through the PNAS open access option. 32 33 34 36 ( S, S, S, and S) for pyrite from the deep-sea sediments of 1To whom correspondence should be addressed. Email: [email protected]. western Canada and Japan. Our study samples from western This article contains supporting information online at www.pnas.org/lookup/suppl/doi:10. Canada were collected at Opal Creek in southwestern Alberta 1073/pnas.1610931114/-/DCSupplemental. 1806–1810 | PNAS | February 21, 2017 | vol. 114 | no. 8 www.pnas.org/cgi/doi/10.1073/pnas.1610931114 Downloaded by guest on September 25, 2021 Fig. 1. Paleogeography for the end-Permian world (∼252 Ma) and the respective location of the Opal Creek and Gujo-Hachiman sections (18, 22). the Permian–Triassic boundary is located near the base of Unit III δ34S/1,000)1.90 – 1). The capital delta notation is defined with expo- (17, 18, 25, 26). nents of 0.515 and 1.90 to approximate the deviation from single- Exact placement of the end-Permian extinction horizon in step low-temperature equilibrium exchange reactions (27, 28). the two study sections allows us to use multiple sulfur isotopes Delta and capital delta values are given in units of per mille (‰). to explore temporal changes of redox chemistry in the Pan- Figs. 2 and 3 present δ34SandΔ33S data of pyrite from the study thalassic Ocean and their potential link to the end-Permian sections (full analytical data of the samples and reference materials mass extinction as well as the following protracted marine available as Tables S1–S3). biotic recovery. Before the end-Permian extinction, δ34S compositions at Opal The multiple sulfur isotope data of pyrite from the Opal Creek Creek vary from –24.97‰ to –10.17‰ with Δ33Sfrom–0.028‰ and Gujo-Hachiman sections are presented using conventional to +0.021‰ (Fig. 2). In the same interval at Gujo-Hachiman, δ34S 3i 3i 32 3i 32 33 delta notation: δ S = 1,000 × (( S/ S)sample/( S/ S)reference ‒ 1), ranges from –40.18‰ to +13.27‰ with Δ Sfrom–0.099‰ to where 3i = 33, 34, or 36. Capital delta notation is also defined to +0.095‰ (Fig. 2). The end-Permian extinction horizon at Opal describe relationships involving the least abundant isotopes: Δ33S = Creek (sample Chang+28) exhibits δ34Sof–23.06‰ and Δ33Sof δ33S − 1,000 × ((1 + δ34S/1,000)0.515 – 1), Δ36S = δ36S − 1,000 × ((1 + –0.017‰ (Fig. 2). The same horizon at Gujo-Hachiman (sample 34 33 34 33 . δ S (‰) Δ S (‰) δ S (‰) Δ S (‰) g. Mb. Fm. Lith. Lith. Con Stag. Sys. Sys. -30 -20 -10 0 -0.04 0.00 0.04 0.08 Sta -50 -30 -10 10 -0.10 0.00 0.10 ic s stone as III Induan Tri Silt a EPME EARTH, ATMOSPHERIC, Veg II AND PLANETARY SCIENCES sic s e Induan Tria ston Conodont Zones Conodont t I mian r e Sulphur Mountain Sulphur P so Sil so o Changhsingian hr P 5m 0.5m 34 EPME 2 P. Ch. 1 shale siltstone chert fine sandstone claystone -30 -20 -10 0 -0.04 0.00 0.04 0.08 -50 -30 -10 10 -0.10 0.00 0.10 Fig. 2. Permian-Triassic biostratigraphy and multiple S-isotopic data for the Opal Creek (Left)andGujo-Hachiman(Right) sections (filled red circles indicate negative Δ33S).
Details
-
File Typepdf
-
Upload Time-
-
Content LanguagesEnglish
-
Upload UserAnonymous/Not logged-in
-
File Pages5 Page
-
File Size-