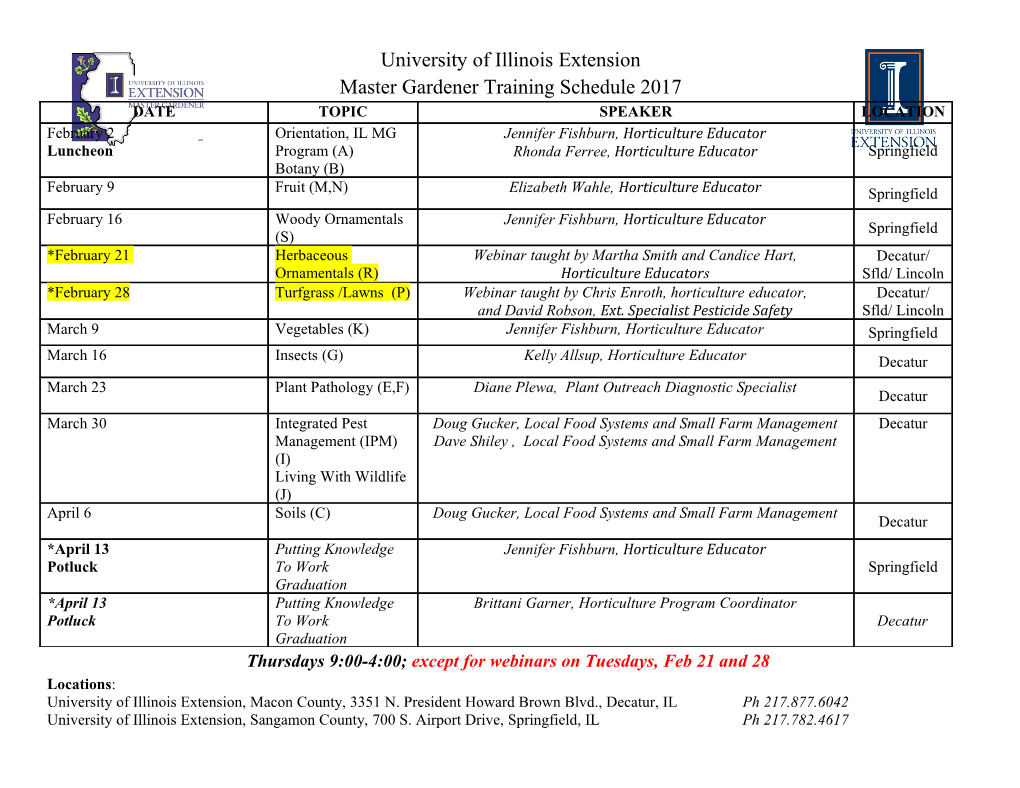
The Pennsylvania State University The Graduate School Department of Engineering Science and Mechanics BIOCHIPS EMPLOYING MICROELECTRODES AND MICROTRANSDUCERS FOR CHARACTERIZATION AND PERMEABILIZATION OF CELL MEMBRANE AT THE WHOLE–CELL AND CELLULAR LEVEL A Dissertation in Engineering Science and Mechanics by Myo Min Thein © 2010 Myo Min Thein Submitted in Partial Fulfillment of the Requirements for the Degree of Doctor of Philosophy December 2010 The dissertation of Myo Min Thein was reviewed and approved* by the following: Jian Xu Associate Professor of Engineering Science and Mechanics and Adjunct Professor of Electrical Engineering Thesis Advisor and Chair of Committee Bernhard R. Tittmann Schell Professor of Engineering Science and Mechanics Yinong Yang Associate Professor of Plant Pathology Sulin Zhang Assistant Professor of Engineering Science and Mechanics Judith A. Todd P. B. Breneman Department Head Chair Head of the Department of Engineering Science and Mechanics * Signatures are on file in the Graduate School. ii Abstract In this study, two types of biochips, Integrated Microelectrode Array (IMA) chip and Ultrasonic Micro–Transducer Array (UMTA) biochip, were designed and micro– fabricated. The IMA chip employs cell–size microelectrode array architecture in order to electrically characterize cellular components, especially the properties of cell membrane, at the single–cell level. The UMTA biochip consists of arrays of high–aspect–ratio piezoelectric micro–transducers that are employed to efficiently permeabilize the cell membrane using ultrasound (i.e., sonoporation) at cellular level with high spatial specificity and resolution. The device fabrication processes, which are tailored towards technology transfer to biochip industries, include laser lithography, proximity and contact photolithography, Physical Vapor Deposition (PVD), high–frequency pulsed electroplating, Chemical Vapor Deposition (CVD) and Plasma–Enhanced Chemical Vapor Deposition (PECVD), furnace annealing and surface passivation, Reactive Ion Etching (RIE) and Inductively–Coupled Plasma (ICP) etching, chemical and mechanical planarization/polishing (CMP), and bottom–up self–assembled monolayer (SAM)– based surface engineering approaches. In demonstrating and testing the performance of these devices, firstly, the electrical characterizations of individual cells (mouse fibroblast (NIH3T3) cell line) were carried out using the IMA biochip integrated with phase–sensitive lock–in detection technique. Bottom–up SAM–based surface engineering approaches were employed to immobilize individual NIH3T3 cells on the working microelectrodes of IMA biochip for the electrical characterizations. The application–specific models such as equivalent electrical circuits of cell–electrode hetero–structures were also developed and cellular properties such as cell membrane capacity, cell membrane resistivity, and average cell–to–substrate separation distance were estimated by numerically fitting the experimental data to the iii developed models. The influence of cell adhesion and spreading, mediated by surface– bound cell adhesion molecules (e.g., fibronectin) or short peptides (e.g., lysine– arginine–glycine–aspartic acid), on the impedance characterizations and sensitivity of cell–based sensors were investigated. In addition, the significances and advantages of single–cell–level impedance measurements in terms of sensitivity in drug screening and toxicity assays were investigated by monitoring real–time cellular changes in individual human glioblastoma (U87MG) cells, seeded on single–cell microelectrodes, upon exposure to an ion channel inhibitor, chlorotoxin (CTX). Results show that microelectrode array architecture combined with proper bioinstrumentations and theoretical models are capable of estimating cellular properties, especially the properties of cell membrane, at the single–cell level as well as monitoring cellular responses to drugs or toxins with high sensitivity, rendering a huge potential of IMA chips in high– throughput cell–based sensing and assays at the single–cell level. Secondly, experiments were carried out to demonstrate the ultrasound–induced site–specific cell membrane permeabilization (or site–specific sonoporation) at the cellular level using the UMTA biochip. The site–specific sonoporation was achieved by applying ultrasonic waves, generated by driving the micro–transducer arrays with high– frequency RF signal generator, onto the targeted cancer cells (human melanoma (LU1205) cell line) within the cell monolayer. Water–soluble Quantum Dots (QD) (carboxylic–acid–surface–derivatized CdSe/ZnS core/shell QDs) were employed as optical probes in order to track the diffusion and transport of nanoparticles across the cell membrane after sonoporation and analyses were carried out by fluorescence microscopy and image processing algorithms. Results show that, above the threshold, moderate ultrasound radiation pressures generated by the micro–transducer arrays can transiently permeabilize the cell membrane at the cellular level with high spatial specificity and resolution. Furthermore, by comparing with the dynamics of iv endocytosis–driven QD intake obtained via flow cytometry and time–lapse fluorescence microscopy, the resulting sonoporation showed significant enhancement in the transport and uptake of water–soluble QDs into the melanoma cells (by an QD–uptake enhancement factor of >80) due to passive diffusion of QDs through membrane wounds. Models representing ultrasound–inflicted cell membrane wounds and mass transport of nanoparticles through wound openings were developed. Model–based analyses, supported with experimental findings, show that low and moderate acoustic radiation pressures generated from the micro–transducer stress the plasma membrane of LU1205 cells and, above the threshold radiation pressure (~0.12MPa for LU1205 cells), large transient wounds are created in the membrane and are actively resealed. The effective size of membrane wounds as well as the uptake of water–soluble QDs were found to be linearly dependent on the ultrasonic pressure applied. These results from site–specific sonoporation show that not only does the UMTA biochips have huge potentials in transfection and gene therapy assays at the cellular level, they also render a promising future as sub–systems in enhancing the delivery efficiency of drug screening and characterization platforms. v TABLE OF CONTENT Abstract.......................................................................................................................... iii List of Figures ..............................................................................................................xv List of Tables ...........................................................................................................xxviii Acknowledgement ...................................................................................................xxix Chapter 1 – Introduction.............................................................................................1 1.1. Motivation...........................................................................................................1 1.2. Objectives and Goals.......................................................................................... 4 1.3. Significance of the Study.....................................................................................5 1.4. Overview of the Dissertation ............................................................................. 6 References ................................................................................................................... 8 Chapter 2 – Cell Membrane and Cell Junctions..................................................10 2.1. General Structure and Functions of Cell Membrane .......................................10 2.2. Cell Membrane Dynamics.................................................................................10 2.2.1. Diffusion across the Cell Membrane .......................................................12 2.2.2. Protein–Mediated Transport and Cell Membrane Potential..................13 2.2.2.1. The Resting Membrane Potential...................................................16 2.2.3. Vesicular Transport .................................................................................16 2.2.4. Transepithelial Transport........................................................................ 17 vi 2.2.5. Osmosis and Tonicity ..............................................................................18 2.3. Cell Junctions....................................................................................................19 2.3.1. Extracellular Matrix................................................................................ 20 2.3.2. Cell–Cell and Cell–Matrix Junctions and Adhesion.............................. 20 2.3.2.1. Cell–Cell Junctions........................................................................ 20 2.3.2.2. Cell–Matrix Junctions................................................................... 23 2.4. Chapter Appendix .............................................................................................25 2.4.1. Diffusion across the Cell Membrane .......................................................25 2.4.1.1. Simple Diffusion .............................................................................25 2.4.1.2. Facilitated Diffusion ...................................................................... 26 2.4.2. Active Transport
Details
-
File Typepdf
-
Upload Time-
-
Content LanguagesEnglish
-
Upload UserAnonymous/Not logged-in
-
File Pages330 Page
-
File Size-