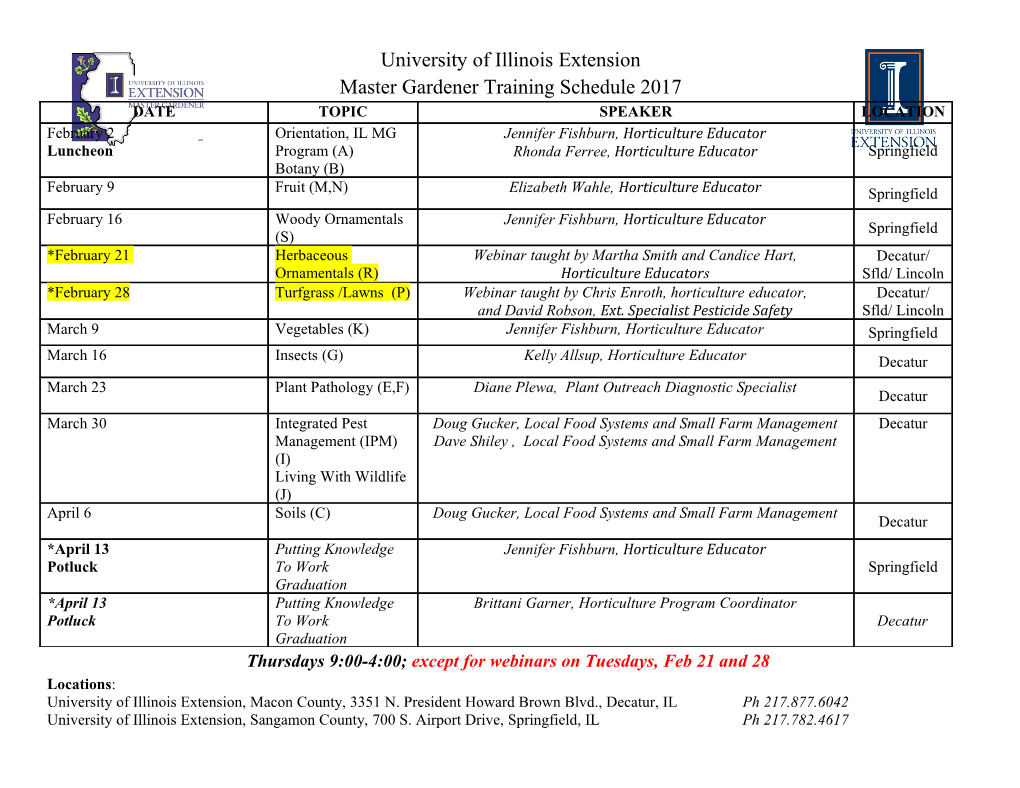
SPECIAL FEATURE: PERSPECTIVE Molecular dynamics and protein function M. Karplus*†§ and J. Kuriyan§¶ʈ *Department of Chemistry and Chemical Biology, Harvard University, Cambridge, MA 02138; †Laboratoire de Chimie Biophysique, Institut de Science et d’Inge´nierie Supramole´culaires, Universite´Louis Pasteur, 67000 Strasbourg, France; ¶Howard Hughes Medical Institute and Departments of Molecular and Cell Biology and Chemistry, University of California, Berkeley, CA 94720-3202; and ʈPhysical Biosciences Division, Lawrence Berkeley National Laboratory, Berkeley, CA 94720 Edited by Bruce J. Berne, Columbia University, New York, NY, and approved February 15, 2005 (received for review January 18, 2005) A fundamental appreciation for how biological macromolecules work requires knowledge of structure and dynamics. Molecular dy- namics simulations provide powerful tools for the exploration of the conformational energy landscape accessible to these molecules, and the rapid increase in computational power coupled with improvements in methodology makes this an exciting time for the ap- plication of simulation to structural biology. In this Perspective we survey two areas, protein folding and enzymatic catalysis, in which simulations have contributed to a general understanding of mechanism. We also describe results for the F1 ATPase molecular motor and the Src family of signaling proteins as examples of applications of simulations to specific biological systems. he conformational dynamics of protein domains that are not expected protein molecules is encoded in to undergo large conformational transi- their structures and is often a tions (e.g., an SH2 domain bound to a critical element of their func- phosphopeptide), a simulation lasting Ttion. A fundamental appreciation for several nanoseconds in a fully solvated how proteins work therefore requires an environment and using all-atom poten- understanding of the connection be- tials without truncation of the electro- tween three-dimensional structure, ob- static interactions will typically result in tained with increasing rapidity by x-ray rms deviations from the crystal struc- crystallography and NMR, and dynam- ture of Ϸ1 Å for the backbone atoms of ics, which is much more difficult to the core region of the protein (7). Such probe experimentally. Molecular dynam- simulations engender a degree of confi- ics simulations provide links between dence that the interesting structural structure and dynamics by enabling the changes that result from the simulation exploration of the conformational en- of larger assemblies [e.g., intact Src ki- ergy landscape accessible to protein nases containing SH2 domains (8)] are molecules (1–3). The first molecular dy- meaningful, as we describe later. namics simulation of a protein was re- Fig. 1. Simulation of a solvated protein. This slice Simulations are most effective when ported in 1977 and consisted of a 9.2-ps through a simulation system shows a Src kinase analyzed in close conjunction with ex- Ϸ trajectory for a small protein in vacuum protein (green) surrounded by 15,000 water mol- periments on protein function, which ecules (oxygen atoms are red and hydrogen atoms (4). Eleven years later, a 210-ps simula- play an essential role in validating and are white). The simulation system consists of improving the simulations (see, for ex- tion of the same protein in water was Ϸ50,000 atoms, including potassium and chloride reported (5), and the phenomenal in- ions (purple and orange spheres, respectively). A ample, refs. 9–12). One outcome of the crease in computing power since then 1-ns molecular dynamics trajectory for this system increase in readily available computer now makes it routine to run simulations can be generated in 4 days by using a cluster of four power and the standardization of simu- of much larger proteins that are 1,000– inexpensive Linux-based computers. (Courtesy of lation protocols is that it is now becoming 10,000 times as long as the original Olga Kuchment.) feasible for nonspecialists to reproduce simulation (Ϸ10–100 ns), in which the and check some, if not all, of the con- protein is surrounded by water and salt clusions of simulation analyses published lations can provide the ultimate detail by others. This kind of cross-validation (Fig. 1). Significant improvements in the concerning individual atomic motions as potential functions have also been will aid in the integration of simulations a function of time; thus, they can be achieved, making the simulations much into the normal process of interpreting used to answer specific questions about more stable and accurate (6). newly emerging structural results. A the properties of a model system often The engineering principles underlying prescription for making the analysis of protein design are truly baroque, with more readily than experiments on the simulation results more rigorous and evolutionary tinkering resulting in molecu- actual system. For many aspects of bi- a list of possible errors has been pro- lar machines that may be effective and omolecule function, these details are of vided (13). efficient but whose three-dimensional interest (e.g., which of the many resi- In this Perspective, we illustrate the structures are often so complicated as to dues surrounding ATP in a motor pro- application of molecular dynamics simu- lations to biology by describing several obscure the mechanism of action. Clues tein are most important for coupling selected examples, emphasizing our own to the essential dynamics of the mole- ATP binding to molecular movement). work. We start with a broader survey of cule are quite often provided by the The combination of increased com- two areas, protein folding and enzymatic separation of the protein into domains puter power and improved potential connected by hinges and the availability functions has resulted in an ability to generate simulations that approach the of structures corresponding to different This paper was submitted directly (Track II) to the PNAS functional states, but it is not easy from point at which they can survive critical office. visual inspection or simple calculations examination by the experimentalists who §To whom correspondence may be addressed. E-mail: to deduce the workings of these molecu- determine the structures of the proteins [email protected] or [email protected]. lar machines. Molecular dynamics simu- being simulated. For small proteins or © 2005 by The National Academy of Sciences of the USA www.pnas.org͞cgi͞doi͞10.1073͞pnas.0408930102 PNAS ͉ May 10, 2005 ͉ vol. 102 ͉ no. 19 ͉ 6679–6685 Downloaded by guest on September 30, 2021 catalysis, in which simulations combined with experiment have led to a general understanding of mechanism. The ex- plosive growth of structural results for an increasingly comprehensive range of biological processes demands simula- tions that address issues that are specific to particular systems. As examples, we describe simulation results for the F1 ATPase molecular motor and the Src family of signaling proteins. Protein Folding An understanding of how the newly syn- thesized polypeptide chain is able to fold to its native structure is fundamen- tal to the description of life at a molecu- lar level. This issue is made all the more critical because misfolding is involved in a range of diseases (14). A synergy be- tween simulation and experiment has led to a conceptual solution for one as- pect of the folding problem that is con- cerned with the mechanism by which the protein chain finds the native conforma- tion despite the immensity of the poten- tial search space. This advance has occurred despite the fact that folding is slow on the simulation time scale. (Even the fastest proteins take microseconds Fig. 2. Simulations of the folding of a three-helix bundle protein. (a and b)(Upper) Semilog plots of the time dependence of the fractions of native helical and interhelical contacts and the inverse fractions of to fold, and the process cannot be simu- native volume (calculated from the inverse cube of the radius of gyration) for two different trajectories. lated directly.) Most of the simulations (Lower) Structures of the protein molecule at selected times. [Reproduced with permission from ref. 20 of folding have used simplified models (Copyright 1999, Nature Publishing Group).] to capture the essential aspects of the process (15–17). Key insights as to how the native One such study of three-helix bundle formation from random coil peptides, structure can be found by the polypep- proteins used a C␣ model to represent illustrating a possible mechanism by tide chain in a reasonable time were the protein chain and a square well po- which large, relatively well ordered obtained by using Monte Carlo dynam- tential for the interactions between pairs -sheet aggregates could appear in solu- ics for the folding of simplified bead of nonbonded residues. These simplifica- tion (23). models on a cubic lattice, a process fast tions made possible the use of discrete It is not yet possible to do statistically enough so that the hundreds of trajecto- molecular dynamics algorithms for meaningful folding simulations of pro- ries needed to obtain statistically mean- studying the folding process (20). The teins with all-atom models. However, ingful results could be calculated with speed of the latter is such that several peptides composed of 20 or so residues the available computers (18). These lat- hundred folding trajectories could be with a well defined native structure are tice Monte Carlo studies
Details
-
File Typepdf
-
Upload Time-
-
Content LanguagesEnglish
-
Upload UserAnonymous/Not logged-in
-
File Pages7 Page
-
File Size-