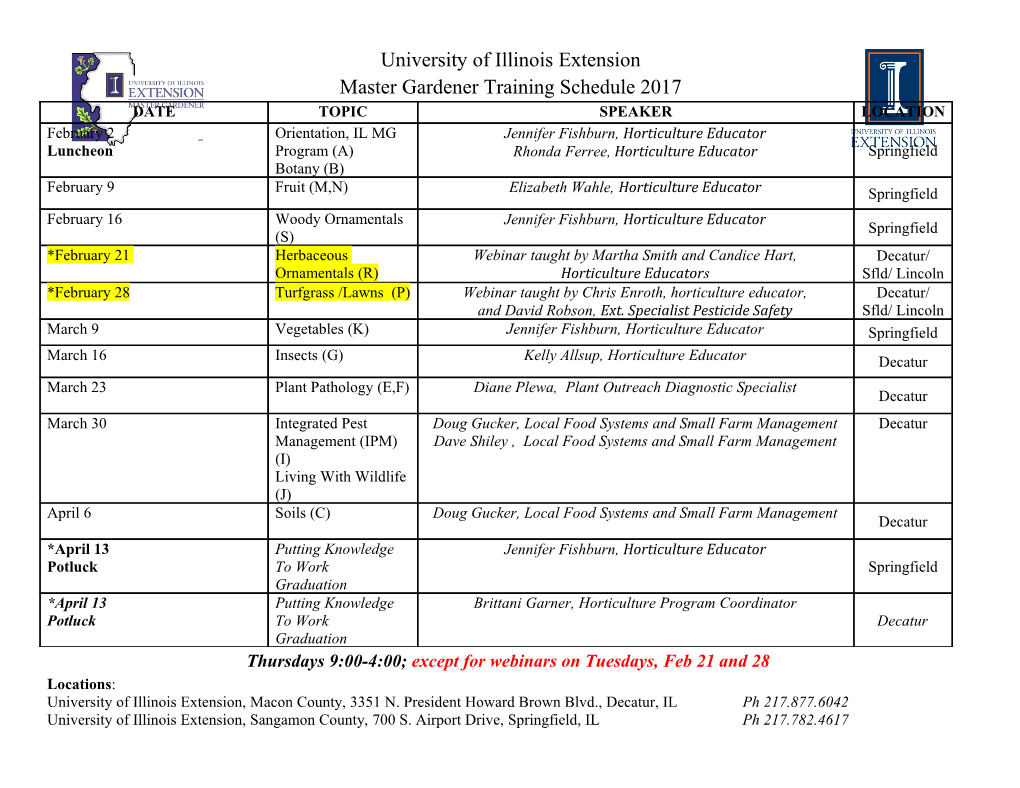
A Dissertation entitled The Transition From Diffuse to Dense Molecular Clouds by Johnathan S. Rice Submitted to the Graduate Faculty as partial fulfillment of the requirements for the Doctor of Philosophy Degree in Physics Dr. Steven R. Federman, Committee Chair Dr. B-G Andersson, Committee Member Dr. Song Cheng, Committee Member Dr. Tom Megeath, Committee Member Dr. John-David Smith, Committee Member Dr. Amanda C. Bryant-Friedrich, Dean College of Graduate Studies The University of Toledo Dec 2018 Copyright 2018, Johnathan S. Rice This document is copyrighted material. Under copyright law, no parts of this document may be reproduced without the expressed permission of the author. An Abstract of The Transition From Diffuse to Dense Molecular Clouds by Johnathan S. Rice Submitted to the Graduate Faculty as partial fulfillment of the requirements for the Doctor of Philosophy Degree in Physics The University of Toledo Dec 2018 The atomic to molecular transitions occurring in the diffuse interstellar gas sur- rounding molecular clouds are affected by the local physical conditions (density and temperature) and the radiation field penetrating the material. Our observations at visible wavelengths of CN, CH, CH+, Ca I, Ca II, and K I absorption from McDonald Observatory and the European Southern Observatory are useful tracers of this gas and provide the velocity component structure needed for analyzing lower resolution, archival ultraviolet observations of CO and H2 absorption from the Far Ultraviolet Spectroscopic Explorer. Additional archival data from ultraviolet measurements of CO and C I absorption were acquired with the Hubble Space Telescope to complement other data sets. A relationship between the column densities of CO and H2 has been known for some time. The break in the slope of the power law relation between CO and H2 column densities corresponds to a change in the photochemistry. Our CO and H2 column density measurements strengthen the results from previous surveys over the ranges corresponding to the transition between diffuse molecular gas to translucent gas. These trends are used in conjunction with those seen in other species like CH and H2 to infer information about the physical conditions in the gas. The derivation of densities can provide additional insight into the correct interpre- tation of trends found in our data and other surveys. We further explore the changing iii environment between diffuse and dense gas by using the column densities and excita- tion temperatures from CO and H2 to determine the gas density. The resulting gas densities from this method are compared to densities inferred from other methods such as CN chemistry or C2 and C I excitation. The total proton gas densities (nH = n(H I)+2n(H2)) allow us to find trends in the combined set of tracers. • Densities from C I and C2 excitation are typically smaller than the densities −3 from other tracers. (nH < 100 cm ) • Larger densities are derived from 12CO(J = 1-0) excitation and are similar to + −3 −3 those from C excitation and CN chemistry. (100 cm < nH < 300 cm ) • The largest densities are derived from 12CO(J = 2-1) excitation and are typically −3 larger than those from CN chemistry. (nH > 300 cm ) Additional kinematic associations of the different tracers with H I,C+, 12CO, 13CO, and C18O emission from the GOT C+ survey extend the combined picture from other tracers and strengthen the characterization of diffuse interstellar gas, including CO dark gas, which is not associated with emission from H I at 21 cm or from CO at 2.6 mm. From the kinematic associations, we find • Diffuse Atomic Clouds have components seen in Ca II, Ca I, H I, and sometimes C+; −3 (nH < 100 cm ) • Diffuse Molecular Clouds (CO Dark Gas) have components seen in C+; K I, CH+; 12 13 −3 −3 CH, or CN but without CO or CO emission; (100 cm ≤ nH ≤ 300 cm ) • Dense Molecular Cloud Envelopes have components seen in 12CO and 13CO emission (with the distinction that clouds with 13CO are denser) and any of the additional −3 −3 species seen in emission or absorption. (300 cm ≤ nH ≤ 1000 cm ) Groupings of sight lines, such as those toward h and χ Persei or Chamaeleon provide a chance for further characterization of the environment. The Chamaeleon Region in particu- lar helps illuminate CO dark gas. This complements other dark gas searches with OH, C+, iv and H I Narrow Self Absorption (HINSA) features. The results described here are the latest effort seeking details of the correspondences revealed by the earlier studies and attempt to weave the results from different methods and tracers into a more complete picture. v This manuscript is dedicated to my family: my dad, Scott Eugene Rice, who has always been my hero, my mom, Robin Diane Rice, who has always guided me through the good and bad times, my brother, Martin Alfred Rice, who left this world too soon, and my wife, Nicole Elizabeth Cappelletty, within who I see my future. Acknowledgments The completion of this Dissertation would not have been possible without the patience and understanding of my advisor, Dr. Steven Federman. His input and patience with the numerous revisions of this Dissertation, and all the work leading up to it, is something for which I will always be thankful. I would also like to thank him for giving me encouragement when I needed it, all the help and understanding during the hard times, and all the advice he has had to repeatedly give me over the years. I have also had the help of many collaborators over the years and this work would not be what it is today without their help: Dr. Paul Goldsmith, Dr. William Langer, Dr. Jorge Pineda, Dr. Nicolas Flagey, Dr. David Lambert, Dr. Adam Ritchey, Justin Brown, Dhruv Sengar, Malinda Bender, and Johnathan Winckowski. I also would like to thank the other members of my committee, Dr. Song Cheng, Dr. Tom Megeath, Dr. JD Smith, and Dr. B-G Andersson, for their support and feedback. I am grateful to Yaron Sheffer for allowing me the use of his program ISMOD. This research made use of the SIMBAD database operated at CDS, France. Some of this work was performed at the Jet Propulsion Laboratory, California Institute of Technology, under contract with NASA. This work was supported in part by NASA Grant NNX10AD80G. Additional support, for the work done by Dhruv Sengar and Malinda Bender in the REU program, came from Grants Phy-1004649 and Phy- 1262810, respectively. I would like to thank my parents for not only their help and support but for inspiring me to pursue my dreams and become the person I am today. Lastly, I vii would like to thank my wife, Nicole, for all the support, understanding, and endless patience you have given me since the day I met you. You have changed my life more than I can possibly express. viii Contents Abstract iii Acknowledgments vii Contents ix List of Tables xiii List of Figures xviii 1 Introduction 1 1.1 ISM Overview . 1 1.2 Chemical Transitions in Diffuse Clouds . 3 1.3 CO Dark Gas . 5 1.3.1 Hydroxyl (OH) . 7 1.3.2 CH emission . 8 1.3.3 H I Narrow Self Absorption . 9 1.4 Observations and Models . 9 1.5 Objectives . 13 2 Observations and Data Reduction 15 2.1 Target Stars . 15 2.2 Observations . 16 2.2.1 McDonald Observations . 20 ix 2.2.2 ESO Observations . 21 2.2.3 FUSE Observations . 22 2.2.4 HST Observations . 24 2.2.5 GOT C+ Observations . 27 2.3 Data Reduction . 30 2.3.1 McDonald Observatory Data Reduction . 30 2.3.2 ESO Data Reduction . 33 2.3.3 Known FUSE Detector Effects . 33 2.3.4 FUSE Data Reduction . 35 2.3.5 HST Data Reduction . 36 2.3.6 GOT C+ Reduction . 36 3 Component Fitting 37 3.1 ISMOD . 38 3.2 Fitting Ground-based Spectra . 39 3.2.1 Comparison with Literature Values . 76 3.2.2 HD 197770 . 86 3.3 Fitting of the H2 Bands . 89 3.3.1 b-Values . 90 3.3.2 Velocity Component Structure . 90 3.3.3 Additional Constraints . 91 3.3.4 H2 Results . 92 3.4 Fitting of the CO Bands . 98 3.4.1 Velocity Structure . 98 3.4.2 12CO Bands from HST . 100 3.4.3 12CO Bands from FUSE . 101 3.4.4 13CO Detections with HST . 106 x 3.4.5 12CO and 13CO Results . 106 3.5 Fitting of Additional Species . 113 3.5.1 C I ................................. 113 3.5.2 C2 ................................. 115 3.5.3 Species for the GOT C+ Project . 119 4 Analysis 131 4.1 Column Density Trends . 131 4.2 Excitation and Density . 133 4.2.1 H2 Excitation Temperatures . 134 4.2.2 CO Excitation Temperatures . 138 4.2.3 C I Excitation Analysis . 149 4.3 Chemistry and Density . 154 4.3.1 CN Chemistry . 154 4.3.2 CH+ like CH Chemistry . 172 4.4 h and χ Persei . 174 4.4.1 CO versus H2 . 174 4.4.2 The CN/CH+ and CH/CH+ Ratios . 178 4.4.3 Discussion . 183 4.5 The 12CO/13CO Isotopic Ratio . 184 4.5.1 Regimes . 185 4.5.2 Density Histograms for 12CO/13CO Sample . 187 4.6 Kinematic Associations of GOT C+ Species . 193 4.6.1 A Component by Component Discussion for G014.8-1.0 . 193 4.6.2 Summaries for Other Pointings . 195 4.6.3 Updated Parallax Distances . 201 xi 5 Discussion 202 5.1 Combined Chemistry Picture .
Details
-
File Typepdf
-
Upload Time-
-
Content LanguagesEnglish
-
Upload UserAnonymous/Not logged-in
-
File Pages402 Page
-
File Size-