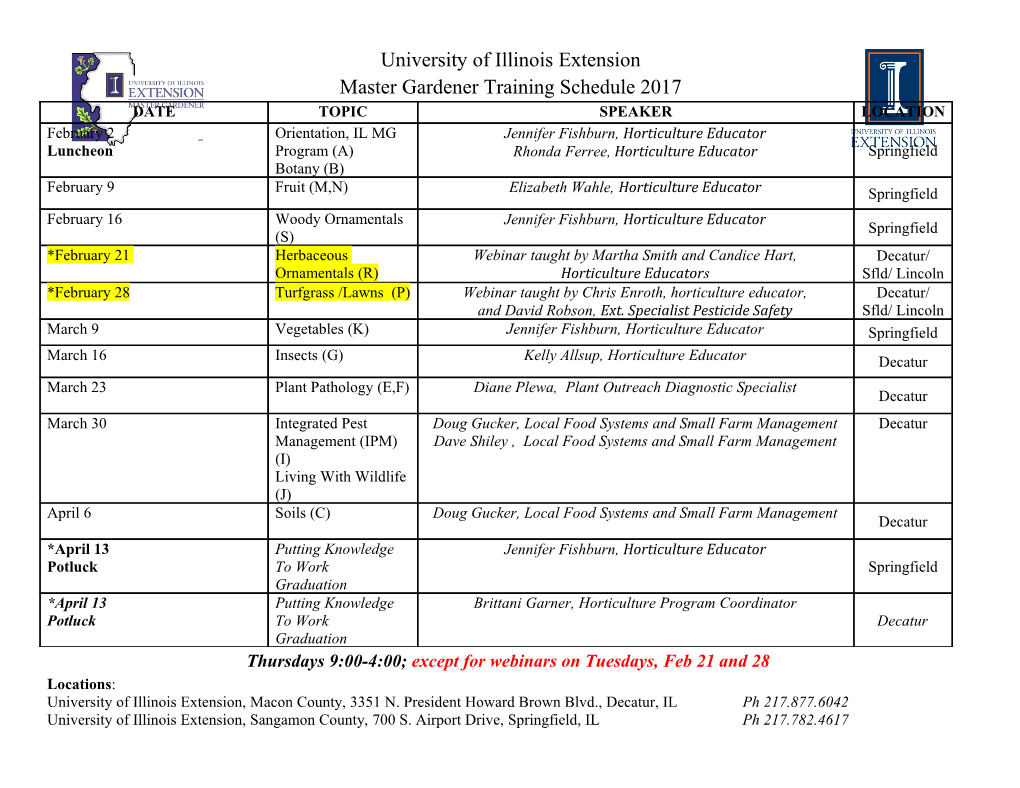
Engineering Analysis for Construction History: Opportunities and Perils John Ochsendorf INTRODUCTION Engineering education, unlike law, philosophy, art, or any field in the humanities, does not require students to learn anything of the history of the profession. Engineering could be compared to medicine, which is a practical field with rapidly developing knowledge and with clear problems to solve. Similar to engineering education, medical students are not required to know the general history of medicine, or even the specific history of a particular disease. Presumably the history of the profession is irrelevant for the practitioners of the future. Fortunately, many engineers over the last century have researched the history of ancient and modern engineering and have made fundamental contributions to the field of construction history. This paper will not attempt to review the accomplishments of engineers in construction history, though the works of Jacques Heyman, Rowland Mainstone, and David Billington can be mentioned as examples of ground-breaking research. In fact, many of the problems facing the historian of construction can be considered to lie within the field of engineering today. The questions faced by historical builders are the problems that today’s engineers attempt to solve. Some of these questions are: • How to build it? • How much does it cost? • How much material is required? • Is it a safe design? • What is the maximum possible span? • What caused the failure of a large structure? All are questions that have been foremost in the minds of builders throughout history. Yet these questions are often neglected by historians of architecture, leaving room for engineers to analyse such historical problems in construction. Though these questions are primarily associated with structural engineering, there are other aspects of engineering which can help to illuminate history, such as material science, construction engineering, manufacturing, etc. This paper explores the role of engineering analysis in construction history and proposes opportunities and pitfalls for the future. In particular, the aim is to demonstrate how structural 89 engineering analysis may provide a new understanding of construction history. Three ongoing research projects will be used as examples to demonstrate the possibilities and to identify potential perils for engineers working on historical topics. Each project is from a different time period and a different region of the world, so they represent a variety of problems in construction history. The three topics are: 1. Early flying buttresses (11th-12th C France) 2. Inca suspension bridges (14th-16th C Peru) 3. Guastavino tile vaulting (19th-20th C United States) These projects are selected because of the author’s experience using engineering analysis to better understand the history of each of these constructions. In each case, the paper will present opportunities and perils for the future. EARLY FLYING BUTTRESSES (11TH-12TH C FRANCE) Working together with Andrew Tallon of Columbia University and Maria-Katerina Nikolinakou of MIT, the author has performed structural studies on early French flying buttresses. The goal was to develop a new understanding of the structure and form of some of the earliest French flying buttresses. As demonstrated by Heyman (1966), such structures can be analysed as an assembly of rigid blocks using limit analysis, in which the minimum and maximum thrust states can be determined from static equilibrium. (fig.1) New research at MIT has created interactive limit analysis tools which can be used to quickly investigate the forces in masonry structures (Block 2005). In particular, the minimum thrust values of flying buttresses are now straightforward to determine. Minimum thrust Maximum thrust Figure 1. Generic flying buttress with minimum and maximum thrust states (after Heyman 1995) Initially, twenty flying buttresses were studied in detail. (fig.2) Andrew Tallon surveyed the buttresses in France to provide the geometry for the structural studies. All buttresses were then 90 analysed to determine the minimum horizontal thrust values and the full results have been published in a French engineering journal (Nikolinakou et al 2005). Despite a wide range in spans and forms of the flying buttresses, most provided a minimum horizontal thrust value between 20% and 40% of the flyer weight. Figure 2. Minimum thrust states of early flying buttresses in France, drawn at the same scale, with the darker line showing the state of minimum thrust (after Nikolinakou et al. 2005) 91 The most significant discovery was that many flyers are in danger of sliding at a state of minimum thrust, as illustrated by the sliding failure limit for a friction coefficient of 0.75. (fig.3) In fact, the surviving flying buttresses appear to cluster on the safe side of the sliding limit. This suggests that trial and error demonstrated this boundary of safe design. As Heyman (1995) has pointed out, measures were sometimes taken to prevent sliding at the head of the flyer, such as the addition of columns under the head of the flyer. For example, the flying buttress at Saint-Julien in Royaucourt, France, has experienced some sliding at the head of the flyer despite the use of a supporting column affixed to the wall. (fig.4) Such movements may have occurred during the initial construction when the flying buttress acted at a state of minimum thrust. Example of shapes: 0.80 8 0.80 0.70 0.70 0.60 6 0.60 Length 0.50 Variable: 0.50 Thickness 0.40 4 Cx 0.40 Cd Flyer geometry R Se 0.30 3 Pc 0.30 Ve Sl PnNc Sg Sliding failure MBNv E Ba Vo 0.20 Friction coefficient: 0.75 SsLc 0.20 Lm Angle 0 102030405060 0 102030405060 Length Flying buttress angle Flying buttress angle Figure 3. a) Minimum horizontal thrust values for flying buttresses with varying length-over-thickness ratios and different inclinations; b) Corresponding thrust values for case study early Gothic flyers There is tremendous potential for such studies to shed new light on the structure and construction of historic masonry monuments. By developing new interactive limit analysis tools, researchers from a wide variety of disciplines will be able to find new answers to old questions. The opportunities for a new understanding of design and construction of masonry architecture can go together with new educational tools for students and professionals. By emphasising static equilibrium as the most important principle, a new level of understanding can emerge among historians of construction. Ongoing work at MIT aims to make such analysis methods more accessible for non-engineers (Block et al. 2006). Simple analysis tools are available for free over the internet for other researchers to use at http://web.mit.edu/masonry. This type of study suggests a number of perils for historians of construction as well. In some cases, engineers are applying modern analysis tools that are not appropriate for historic structures. First 92 and foremost is the use of elastic analysis for masonry structures. As an example, consider the two semicircular arches of Figure 5, one with a thickness/radius ratio of 8% (fig.5a) and the other with a ratio of 16% (fig.5b). A typical finite element analysis is shown on the left, in which the resulting elastic stress patterns in the masonry are indistinguishable between the two arches (Block et al 2006). Yet, a simple thrust line analysis at right immediately shows the difference between the two structures. The 8% arch will not stand unless the material can withstand considerable tension, which is not the case for historic masonry construction. In short, elastic analysis says nothing about the collapse state of masonry and cannot be used to describe the actual state of a historic masonry structure. Unfortunately, many engineers are applying elastic analysis to unreinforced masonry, which generally does not offer useful information for the historian. Elastic finite element analysis, which purports to find the “one true state of a structure”, is seeking exactness in vain (Ochsendorf 2005). Figure 4. Sliding near the support of a flying buttress at Saint-Julien in Royaucourt, France (Photo: Andrew Tallon) This study of flying buttresses suggests another peril facing engineers. Without proper historical training, an engineer may tend to adopt an evolutionary view of history, in which some designs are “better” or more “efficient.” To apply such an idea to the early French flying buttresses would be fruitless, because without additional archaeological or historical knowledge, it would be difficult to draw strong conclusions on the evolution of flying buttress designs. The desire to look for evolution based on engineering analysis is tempting, but should only be undertaken with the appropriate 93 historical and archaeological research in support of the engineering analysis. In modern engineering, there is a prevalent notion that each generation of engineers will surpass the previous generation in a constant march of progress. With new technology (such as faster computers) engineering will always move forward to a more enlightened state of knowledge. Such notions are dangerous for modern engineering, and can be even more dangerous when applied to history. Figure 5. Two semicircular stone arches of varying thickness a) 8% and b) 16% are analysed by finite elements (at left) and by thrust lines using limit analysis (at right). (Block et al. 2006) The example of flying buttresses has illustrated how the use of simple equilibrium analysis can shed new light on the performance and construction of historic masonry structures. While this example has illustrated how engineering analysis can offer greater understanding of a specific element of a masonry building, the next example suggests that engineers can uncover wider societal questions that have been neglected by historians. INCA SUSPENSION BRIDGES (14TH-16TH C PERU) The history of ancient suspension bridges is a fascinating topic which has scarcely been explored. In ancient China, iron chain suspension bridges spanned long distances more than 1,000 years before the iron bridge at Coalbrookdale in 1779, which is often hailed as the first metal structure.
Details
-
File Typepdf
-
Upload Time-
-
Content LanguagesEnglish
-
Upload UserAnonymous/Not logged-in
-
File Pages22 Page
-
File Size-