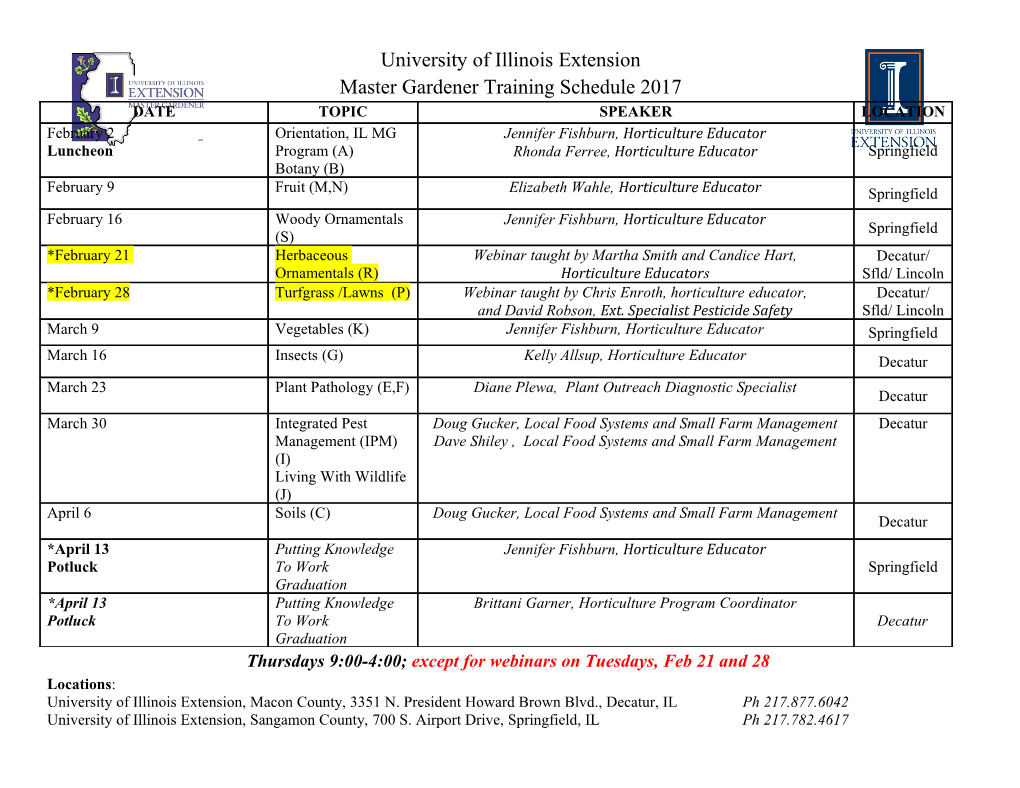
Dark Matter Searches and Study of Electrode Design in LUX and LZ Adam Bailey Imperial College London Department of Physics A thesis submitted for the degree of Doctor of Philosophy August 2016 Abstract There is substantial evidence that over 80% of matter in the universe is dark matter – which is non-baryonic in nature and is thought to be composed of a new, slow-moving, stable particle not found in the Standard Model of Particle Physics. Its presence is inferred from gravitational effects on luminous matter from several independent ob- servations, from the galactic to the cosmological scale. Weakly Interacting Massive Particles (WIMPs) are the leading candidate, which can explain all of the observed effects. LUX and LZ are dual-phase xenon time projection chambers (TPC), aiming to ob- serve scattering of WIMPs from xenon nuclei. LUX has an active mass of 250 kg of liquid xenon, and took data at the Sanford Underground Research Facility in Lead, South Dakota, between 2013 and 2016. The first WIMP search run of 85 live days in 2013 set world-leading exclusion limits on the spin-independent WIMP-nucleon cross section. This was improved by a reanalysis of those data, and subsequently by a new run yielding 332 live days, which set a minimum exclusion limit of 2.2 10 46 cm2 × − for a 50 GeV WIMP (90% CL). In addition, the most stringent limit to date on the spin- dependent WIMP-neutron scattering cross section comes from the reanalysis of the 2013 dataset, with a minimum exclusion of 9.4 10 41 cm2 for a 33 GeV WIMP. LZ is × − a next generation experiment with a 7 tonne active mass to be deployed in the same location as LUX, expected to be 100 times more sensitive. Work presented in this thesis includes analysis of the 2013 LUX search data to pro- duce the spin-dependent results, evaluating the detector response using a tritium β− source, and determining the 85Kr background from data. A study was carried out on spurious electron emission phenomena from thin cathodic wires under high elec- tric fields, using LUX engineering data where the grid voltages were increased above nominal operating values; this led to new insights into the microscopic breakdown mechanisms which have affected these (and other) TPC detectors for decades. The de- tailed understanding of the electroluminescence response gained in LUX was applied to the design of the LZ electroluminescence region; detailed simulation work of elec- trode geometry was performed to assess the performance of several candidate designs. Declaration I declare that material in this thesis is my own work. Any work that is not my own is appropriately stated and referenced. The specific contributions in each chapter are listed below. Chapters 2 and 3 are describing background information on dark matter and the LUX detector. The latter describes work by the LUX and LZ collaborations. Run 3 of the LUX experiment is described in Chapter 4. My contributions were the development of a real time data monitor, an analysis to determine the detection efficiency from tritium data, and a 85Kr background analysis. The section on the WIMP search analysis in Chapter 4 is describing collaboration work. For Chapter 5 I implemented the spin-dependent calculations into the existing LUX analysis code, and assisted in developing the code further. I also assessed the im- pact of nuisance parameters on the result. The section on the signal estimation de- scribes the statistical test used in the analysis code. The sections on the background models, detector response simulations and the LUX data analysis are describing work done by the collaboration. I was responsible for producing the final results on the spin-dependent scattering cross sections. The exclusion regions on the WIMP-proton and WIMP-neutron coupling constants were done with assistance from Nicole Larsen (Yale). The study of electron emission phenomena presented in Chapter 6 was an indepen- dent analysis. The data used were taken by collaborators during the LUX conditioning campaign. The simulations in Chapter 7 were done in collaboration with Pawel Majewski (RAL), who provided assistance with the simulation software and creation of the elec- tric field maps. This included creating the original field map for the woven wire mesh and the XENON100 grids. The rest of the simulation work in this chapter was my own. – Adam Bailey The copyright of this thesis rests with the author and is made available under a Cre- ative Commons Attribution Non-Commercial No Derivatives licence. Researchers are free to copy, distribute or transmit the thesis on the condition that they attribute it, that they do not use it for commercial purposes and that they do not alter, transform or build upon it. For any reuse or redistribution, researchers must make clear to others the licence terms of this work. Acknowledgements Firstly, I would like to thank my supervisor Henrique Araujo´ for invaluable guidance and assistance throughout my PhD and thesis writing. He was always there with use- ful advice and discussion for any aspect of my studies, and I feel that I have benefited greatly from his experience. I extend my thanks to coworkers who have assisted me in various ways. To Pawel Majewski for the help with the simulation software for the LZ design work, along with useful feedback on that chapter. Also to Thomas Shutt and Jerry Va’vra for feedback and discussion on that same work. To Daniel McKinsey, Wing To, Nicole Larsen, Alas- tair Currie, and Aaron Manalaysay for their assistance and input on the LUX Run 3 reanalysis. To Attilla Dobi and Matthew Szydagis for useful discussions relating to NEST and tritium, and to Alex Lindote for making the LUX data processing more transparent. To Alfredo Tomas, I learnt many things during the many hours in the lab, although I never want to deal with 50 µm wires again. My gratitude extends to all members of LUX and LZ, both past and present. It is combined effort of many people that has made the success of the experiments possible. It has been a pleasure to work as part of both collaborations. I also wish to acknowledge STFC for funding my studies at Imperial College. There are many people I would like to thank for making the time on-site in South Dakota enjoyable, whether it be eating, drinking, hiking, board games, being stuck in the snow, or even underground in the lab: Doug Tiedt, Mark Hanhardt, Matthew Szydagis (additionally for providing N64), Dev Ashish Khaitan, Claudio Silva, Alex Lindote, Luiz de Viveiros, Carmen Carmona, Jim Dobson, Nicole Larsen, Attilla Dobi, Richard Knoche, Mongkol Moongweluwan, Chris Chiller, Angela Chiller, Curt Nehrkorn (where does the krypton go?), Sally Shaw, James Verbus, Kevin O’Sullivan, Elizabeth Boulton, Markus Horn (Forgive me if I have left anyone out). I actually miss it there sometimes! Lastly, I would like to thank my parents, who have always supported and encour- aged me in whatever I wanted to do. And to Mar´ıa, who has been there for support throughout my PhD, and has probably learnt a fair amount about dark matter herself. Contents 1 Introduction 21 2 Dark Matter – Evidence, Candidates, and Detection 24 2.1 Evidence . 24 2.1.1 Galactic Scale . 24 2.1.2 Cosmological Scales . 27 2.2 Candidates . 30 2.2.1 Weakly Interacting Massive Particles (WIMPs) . 31 2.2.2 Cosmological properties of WIMPs . 32 2.2.3 Non-WIMP particle dark matter . 33 2.3 Detection . 33 2.3.1 Collider searches . 34 2.3.2 Indirect searches . 36 2.3.3 Direct detection . 38 2.3.4 Complementarity of detection methods . 42 3 Direct Detection with Dual-Phase Xenon 44 3.1 Particle Detection in Liquid Xenon TPCs . 44 3.1.1 Prompt scintillation signal . 46 3.1.2 Electron transport and cross-phase extraction . 47 3.1.3 Secondary scintillation light . 48 3.1.4 Light yield, charge yield, discrimination and energy . 49 3.2 The Large Underground Xenon (LUX) Experiment . 51 3.2.1 TPC and cryostat . 52 3.2.2 Grids and high voltage delivery . 53 3.2.3 Photomultipliers . 53 9 10 CONTENTS 3.2.4 Gas system, circulation and purification . 54 3.2.5 Cryogenics and thermosyphon cooling . 55 3.2.6 Instrumentation and slow control . 56 3.2.7 DAQ and electronics . 57 3.2.8 Calibration systems . 58 3.2.9 Water tank and veto . 60 3.3 The LUX-ZEPLIN (LZ) Experiment . 60 3.3.1 The LZ anti-coincidence systems . 62 4 LUX Run 3 64 4.1 Operations and Data Processing . 64 4.1.1 Detector operations . 64 4.1.2 Data processing . 65 4.2 Real Time Data Monitoring . 67 4.2.1 Event and pulse selection . 69 4.2.2 Output and uses . 70 4.3 Calibrations . 74 4.3.1 Krypton-83m . 74 4.3.2 Tritium . 75 4.4 85Kr Background Analysis . 83 4.4.1 Introduction . 83 4.4.2 S1 energy calibration . 85 4.4.3 S1 and S2 energy calibration . 87 4.4.4 85Kr search analysis . 91 4.4.5 S1-only analysis . 92 4.4.6 Combined energy analysis . 97 4.4.7 Conclusions from 85Kr background analysis . 102 4.5 Run 3 WIMP Search Analysis . 102 4.5.1 Backgrounds . 104 CONTENTS 11 4.5.2 Results . 106 4.6 Conclusions . 107 5 Spin-Dependent Limits from the 2015 LUX Run 3 Reanalysis 108 5.1 Spin-Dependent Interaction . 108 5.1.1 Nuclear spin structure . 109 5.1.2 SD WIMP recoil spectrum . 114 5.2 Signal Estimation . 120 5.3 Models for the LUX PLR .
Details
-
File Typepdf
-
Upload Time-
-
Content LanguagesEnglish
-
Upload UserAnonymous/Not logged-in
-
File Pages272 Page
-
File Size-