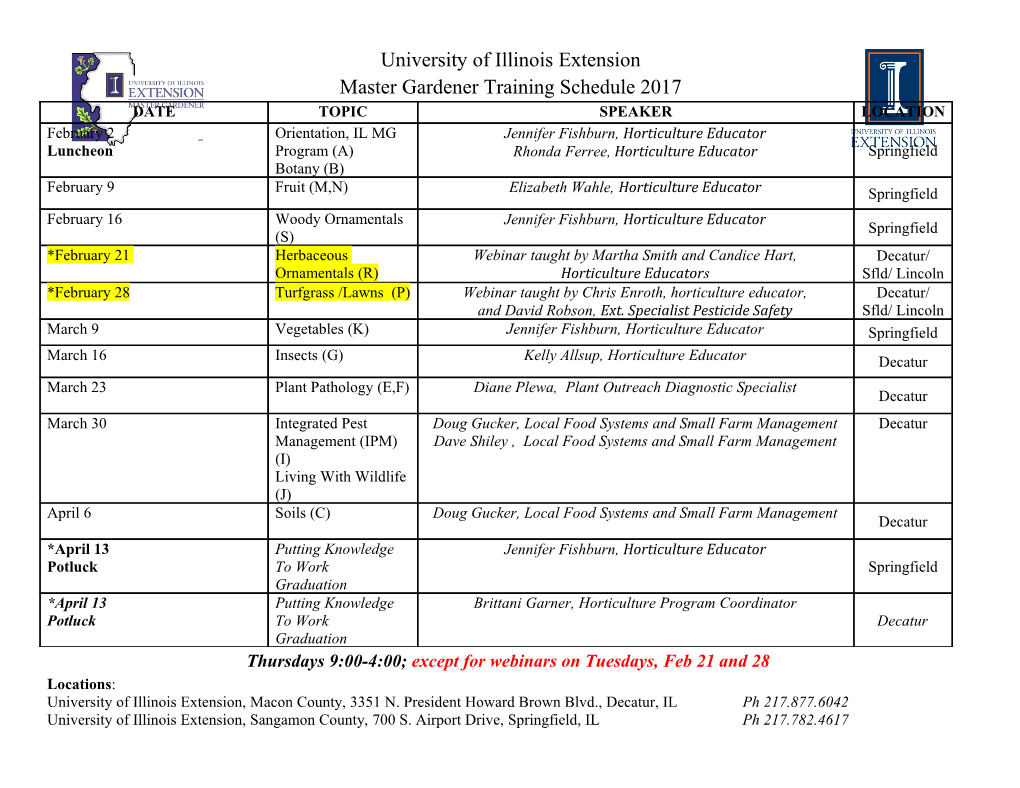
Techniques in molecular spectroscopy: from broad bandwidth to high resolution by Kevin C. Cossel B.S., California Institute of Technology, 2007 A thesis submitted to the Faculty of the Graduate School of the University of Colorado in partial fulfillment of the requirements for the degree of Doctor of Philosophy Department of Physics 2014 This thesis entitled: Techniques in molecular spectroscopy: from broad bandwidth to high resolution written by Kevin C. Cossel has been approved for the Department of Physics Prof. Jun Ye Prof. Eric Cornell Date The final copy of this thesis has been examined by the signatories, and we find that both the content and the form meet acceptable presentation standards of scholarly work in the above mentioned discipline. Cossel, Kevin C. (Ph.D., Chemical Physics) Techniques in molecular spectroscopy: from broad bandwidth to high resolution Thesis directed by Prof. Jun Ye This thesis presents a range of different experiments all seeking to extended the capabilities of molecular spectroscopy and enable new applications. The new technique of cavity-enhanced di- rect frequency comb spectroscopy (CE-DFCS) provides a unique combination of broad bandwidth, high resolution, and high sensitivity that can be useful for a wide range of applications. Previous demonstrations of CE-DFCS were confined to the visible or near-infrared and operated over a lim- ited bandwidth: for many applications it is desirable to increase the spectral coverage and to extend to the mid-infrared where strong, fundamental vibrational modes of molecules occur. There are sev- eral key requirements for CE-DFCS: a frequency comb source that provides broad bandwidth and high resolution, an optical cavity for high sensitivity, and a detection system capable of multiplex detection of the comb spectrum transmitted through the cavity. We first discuss comb sources with emphasis on the coherence properties of spectral broadening in nonlinear fiber and the development of a high-power frequency comb source in the mid-infrared based on an optical-parametric oscillator (OPO). To take advantage of this new mid-infrared comb source for spectroscopy, we also discuss the development of a rapid-scan Fourier-transform spectrometer (FTS). We then discuss the first demonstration of CE-DFCS with spectrally broadened light from a highly nonlinear fiber with the application to measurements of impurities in semiconductor manufacturing gases. We also cover our efforts towards extending CE-DFCS to the mid-infrared using the mid-infrared OPO and FTS to measure ppb levels of various gases important for breath analysis and atmospheric chemistry and highlight some future applications of this system. In addition to the study of neutral molecules, broad-bandwidth and high-resolution spectra of molecular ions are useful for astrochemistry where many of the observed molecules are ionic, for + studying molecules such as CH5 with highly non-classical behavior, and for tests of fundamental iv physics. We have developed a new technique { frequency comb velocity-modulation spectroscopy { that is the first system to enable rapid, broadband spectroscopy of molecular ions with high resolution. We have demonstrated the ability to record 150 cm−1 of spectra consisting of 45,000 points in 30 minutes and have used this system to record over 1000 cm−1 of spectra of HfF+ in the near-infrared around 800 nm. After improvements, the system can now cover more than 3250 cm−1 (700-900 nm). We have combined this with standard velocity-modulation spectroscopy to measure and analyze 19 ro-vibronic bands of HfF+. These measurements enabled precision spectroscopy of trapped HfF+ for testing time-reversal symmetry. For this experiment, we perform Ramsey spectroscopy between spin states in the 3 metastable ∆1 level to look for a permanent electric dipole moment of the electron with what we believe is the narrowest line observed in a molecular system (Fourier limited with 500 ms of coherence time). The long coherence time is a major advantage of using ions, but there are also some added complexities. We discuss various aspects metastable state preparation, state detection, and spectroscopy in a rotating frame (due to the necessary rotating electric bias field) that were particular challenging. In addition, we discuss limits to the coherence time { in particular, ion-ion collisions { as well as the sensitivity of the current measurements and provide a path towards a new limit on the electric dipole moment of the electron. Dedication To my dad, who was an amazing role model and always encouraged my interest in science. In some way, he probably always knew that I would achieve this. vi Acknowledgements None of this work would have been possible without a lot of help from a great group of people. Both Jun and Eric have been great advisors and have tried to teach my how to think like a physicist. If I only succeed in learning a fraction of this, it will still serve me very well! The members of both Jun and Eric's labs are all extremely talented and it has been a pleasure to work with them. I can't hope to name all of the people who I taught me various tips or who lent me critical optics but I would like to thank in particular Mike Martin, Mike Thorpe, Dylan Yost, Arman Cing¨oz,Tom Alison, Florian Adler, Matt Swallows, Craig Benko, Piotr Maslowski, Alexsandra Foltynowicz, Bryce Bjork, AJ Fleisher, Laura Sinclair, Dan Gresh, Huanqian Loh, Matt Grau, and Will Cairncross. I wish the best of luck to them and have no doubt that they will all be extremely successful. In addition to all of the graduate students and postdocs in the labs, the JILA staff that I worked with in the electronics shop (Terry, James, Carl) and instrument shop (Hans, Kim, Tracy, Blaine) are all indispensable for all of the experiments. In addition, Pam, Diane, Krista, Lauren, and all of the other staff do a great job keeping everything running smoothly. Outside of JILA, I am grateful to Scott Diddams and Nate Newbury at NIST for many interesting discussions, Axel Ruehl, Ingmar Hartl and the crew at IMRA for help with lasers, Ronald Holzwarth, Tobias Wilken, Thomas Udem and others at MPQ for hosting me for a month, and Mitchio Okumura and Thinh Bui at Caltech for great collaborations. My parents have provided amazing support and always gave me opportunities to learn something new. I would also than Mike Martin, Carly Donahue, Laura Sinclair, and Galen O'Neil for many fun times outside of the lab. Last, but definitely not least, my wife Eleanor has provided immeasurable support, encouragement, and joy. Contents Chapter 1 Introduction 1 2 Frequency comb sources 9 2.1 Mode-locked lasers . 10 2.1.1 Mode-locking Mechanisms . 12 2.2 Indirect Sources . 15 2.2.1 cw-laser Based Sources . 18 2.2.2 Typical comb sources . 19 2.3 Nonlinear fiber optics . 22 2.3.1 Numerical simulations . 25 2.3.2 Broadening mechanisms . 28 2.3.3 Coherence measurements . 36 2.4 Optical parametric oscillators . 43 3 Detection techniques 57 3.1 Cavity-comb coupling . 57 3.1.1 Tight locking scheme . 59 3.1.2 Swept coupling scheme . 60 3.2 Detection sensitivity . 62 3.3 Detection techniques . 66 viii 3.3.1 VIPA . 67 3.3.2 Fourier-transform spectrometer . 75 3.4 Velocity-modulation spectroscopy . 83 3.4.1 Background . 85 3.4.2 Comb-vms . 87 3.4.3 System performance . 91 3.4.4 Single-frequency vms . 93 3.4.5 Extensions of comb-vms . 98 4 Applications of DFCS 100 4.1 Trace detection in Arsine . 101 4.1.1 Experimental Setup . 103 4.1.2 Data Analysis . 107 4.1.3 Results . 108 4.2 Mid-infrared comb spectroscopy . 114 4.2.1 Measurement of individual molecular species . 116 4.2.2 Instrument performance limits . 117 4.2.3 Multi-line fitting advantage . 120 4.2.4 Determination of absolute concentrations of a gas mixture . 121 4.3 Future applications . 122 4.4 Conclusions . 132 5 Velocity-modulation spectroscopy of HfF+ 133 5.1 Results . 134 5.1.1 Diatomic molecular spectra primer . 134 5.1.2 Fitting . 146 5.1.3 Lambda doubling . 156 5.2 Theory . 160 ix 5.3 Summary . 162 6 Precision spectroscopy of trapped ions 171 6.1 eEDM Background . 171 6.1.1 Measurement Basics . 175 6.1.2 Current Limit . 178 6.1.3 Overview of JILA eEDM measurement . 180 6.2 Experimental Setup . 182 6.2.1 Ion Trap . 184 6.2.2 Photodissociation . 192 6.2.3 Rotating bias fields . 199 6.3 Coherent Transfer . 203 6.3.1 Laser stabilization . 206 6.3.2 Transfer theory . 212 6.3.3 Transfer parameters . 213 6.3.4 Transfer efficiency and timescale . 218 6.3.5 Transfer spectrum . 219 6.4 Ramsey Spectroscopy . 225 6.4.1 Sensitivity Estimates . 232 6.5 Coherence Time . 233 6.5.1 Lifetime . 233 6.5.2 Field inhomogeneity . 236 6.5.3 Collisions . 237 6.5.4 Collision background . 239 6.5.5 Coherence time from collisions . 241 6.6 Systematic Errors . 249 6.6.1 Non-reversing magnetic field . 250 x 6.6.2 Perpendicular magnetic fields . 253 6.6.3 Field inhomogeneity . 254 6.6.4 Other effects . 255 6.7 eEDM measurement . ..
Details
-
File Typepdf
-
Upload Time-
-
Content LanguagesEnglish
-
Upload UserAnonymous/Not logged-in
-
File Pages319 Page
-
File Size-