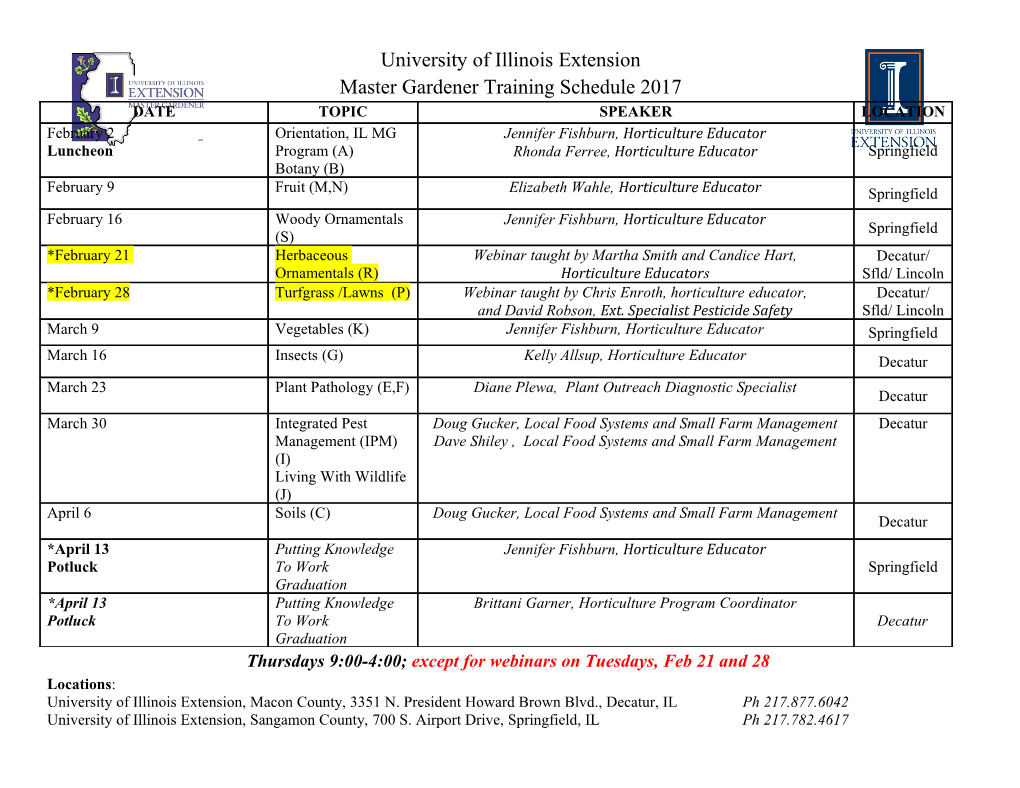
JID:EPSL AID:14933 /SCO [m5G; v1.232; Prn:5/03/2018; 11:04] P.1(1-13) Earth and Planetary Science Letters ( ) – ••• •••• ••• ••• 1Q1 Contents lists available at ScienceDirect 67 2 68 3 69 4 Earth and Planetary Science Letters 70 5 71 6 72 7 www.elsevier.com/locate/epsl 73 8 74 9 75 10 76 11 77 12 Controls on the global distribution of contourite drifts: Insights from 78 13 an eddy-resolving ocean model 79 14 80 15Q2 Amanda C. Thran a, , Adriana Dutkiewicz a, Paul Spence b,c, R. Dietmar Müller a,d 81 16 ∗ 82 17 a EarthByte Group, School of Geosciences, University of Sydney, Sydney NSW 2006, Australia 83 18 b Climate Change Research Centre, University of New South Wales, Sydney NSW 2052, Australia 84 c 19 ARC Centre of Excellence for Climate System Science, University of New South Wales, Sydney NSW 2052, Australia 85 d Sydney Informatics Hub, University of Sydney, Sydney NSW 2006, Australia 20 86 21 87 22 a r t i c l e i n f o a b s t r a c t 88 23 89 24 90 Article history: Contourite drifts are anomalously high sediment accumulations that form due to reworking by bottom 25 91 Received 6 December 2017 currents. Due to the lack of a comprehensive contourite database, the link between vigorous bottom Received in revised form 25 February 2018 26 water activity and drift occurrence has yet to be demonstrated on a global scale. Using an eddy- 92 Accepted 27 February 2018 27 resolving ocean model and a new georeferenced database of 267 contourites, we show that the global 93 Available online xxxx 28 distribution of modern contourite drifts strongly depends on the configuration of the world’s most 94 Editor: M. Frank 29 powerful bottom currents. Bathymetric obstacles frequently modify flow direction and intensity, imposing 95 30 Keywords: additional finer-scale control on drift occurrence. Mean bottom current speed over contourite-covered 96 31 contourite areas is only slightly higher (2.2 cm/s) than the rest of the global ocean (1.1 cm/s), falling below proposed 97 sediment drift thresholds deemed necessary to re-suspend and redistribute sediments (10–15 cm/s). However, currents 32 98 ocean modelling fluctuate more frequently and intensely over areas with drifts, highlighting the role of intermittent, 33 99 meridional overturning circulation high-energy bottom current events in sediment erosion, transport, and subsequent drift accumulation. 34 eddy 100 We identify eddies as a major driver of these bottom current fluctuations, and we find that simulated 35 bottom current 101 bottom eddy kinetic energy is over three times higher in contourite-covered areas in comparison to 36 102 the rest of the ocean. Our work supports previous hypotheses which suggest that contourite deposition 37 predominantly occurs due to repeated acute events as opposed to continuous reworking under average- 103 38 intensity background flow conditions. This suggests that the contourite record should be interpreted in 104 39 terms of a bottom current’s susceptibility to experiencing periodic, high-speed current events. Our results 105 40 also highlight the potential role of upper ocean dynamics in contourite sedimentation through its direct 106 41 influence on deep eddy circulation. 107 42 © 2018 Elsevier B.V. All rights reserved. 108 43 109 44 110 45 111 46 112 1. Introduction between bottom current activity and contourite drift occurrence 47 113 can be difficult to demonstrate in situ for all cases; these fea- 48 114 tures are often highly inaccessible, and investigators have had to 49 Contourite drifts (or “sediment drifts”) are anomalously high 115 rely on sparse current meter measurements or oceanographic tran- 50 accumulations of deep-sea sediment that are largely found around 116 sects to gauge the regional hydrodynamic setting of their survey 51 prominent bathymetric obstacles. These features have become fre- 117 52 quent ocean drilling targets, as they can preserve high-resolution area (Rebesco et al., 2014). Such methods, though essential for 118 53 sedimentological evidence of major paleoceanographic and/or pa- ground-truthing, may not adequately represent the oceanographic 119 54 leoclimatic change (Rebesco et al., 2014). In a series of seminal processes that lead to drift formation. The use of ocean circula- 120 55 papers, Heezen et al. (1966)were among the first to propose tion models can help address these shortcomings, as they simulate 121 56 bottom currents as the main driver for their formation, and the these processes on larger scales while abiding by the physical re- 122 57 link between contourite drifts and the world’s most powerful bot- strictions imposed by fluid dynamics. 123 58 tom currents steadily became apparent as more contourite drifts In bridging the gap between physical oceanography and the 124 59 were discovered (Hollister and Heezen, 1972). However, causality deep-sea sedimentological record, it is becoming more com- 125 60 mon to present simulation results in tandem with site survey 126 61 data (e.g., seismic reflection profiling, core analyses, bathymetry 127 62 * Corresponding author. data, backscatter intensities, current meter measurements, etc.) 128 63 E-mail address: thran @sydney.edu .au (A.C. Thran). as an additional independent line of evidence used to demon- 129 64 130 https://doi.org/10.1016/j.epsl.2018.02.044 65 131 0012-821X/© 2018 Elsevier B.V. All rights reserved. 66 132 JID:EPSL AID:14933 /SCO [m5G; v1.232; Prn:5/03/2018; 11:04] P.2(1-13) 2 A.C. Thran et al. / Earth and Planetary Science Letters ( ) – ••• •••• ••• ••• 1 strate a given contourite’s formation mechanisms (Chen et al., to reach a dynamically steady state. The atmospheric forcing is 67 2 2016;Hanebuth et al., 2015;Hernández-Molina et al., 2011; prescribed from version 2 of the Coordinated Ocean–ice Reference 68 3 Uenzelmann-Neben et al., 2016). Interestingly, numerical simu- Experiments (CORE) data (Griffies et al., 2009). The model accu- 69 4 lations have been used to resolve and investigate lesser-known rately represents global bathymetric features and realistically sim- 70 5 oceanographic processes (e.g., internal waves and dense shelf wa- ulates the critical drivers of bottom flows (e.g., global meridional 71 6 ter cascading) that could be responsible for the formation of many overturning circulation – MOC, wind-driven shallow-water circula- 72 7 shallow-water, smaller-scale drifts and their associated bedforms tion, eddy transport, etc.) associated with sediment drift formation 73 8 (Bonaldo et al., 2016;Droghei et al., 2016; Martorelli et al., 2010; (Rebesco et al., 2014). 74 9 Stow et al., 2009). Nevertheless, a numerical approach to drift oc- Simulated bottom current metrics (e.g., time-mean and max- 75 10 currence has only been implemented on a regional scale (Bonaldo imum current speeds, and speed standard deviation) were then 76 11 et al., 2016;Chen et al., 2016;Droghei et al., 2016;Hanebuth examined in relation to the distribution of contourite drifts. Sim- 77 12 et al., 2015;Haupt et al., 1994;Hernández-Molina et al., 2011; ulated eddy kinetic energy in the model’s bottom layer was also 78 13 Martorelli et al., 2010;Salles et al., 2010), and regional simula- considered, where eddy kinetic energy was computed by decom- 79 14 tions are accompanied by their own set of limitations. Regional posing daily velocity field outputs into their mean and eddy com- 80 15 computational domains can produce boundary artefacts (Haupt et ponents, following the methods of Stewart et al. (2017). For each 81 16 al., 1994)and generally have trouble realistically representing crit- metric, values were extracted from all contourite-covered areas 82 17 ical global-scale processes (e.g., Atlantic meridional overturning (i.e., points that lie within the bounds of the contourite polygons) 83 18 circulation – AMOC) that are thought to exert first-order con- using a Hierarchical Equal Area isoLatitude Pixelization (HEALPix) 84 19 trol on contourite drift distribution throughout the world’s oceans mesh of similar resolution to the computational domain at the 85 20 (Rebesco et al., 2014). To date, the absence of a global, cohesive equator (Gorski et al., 2005). Extraction of these bottom current 86 21 contourite database has prevented the link between large-scale metrics was repeated for all points that comprise the global ocean. 87 22 ocean circulation patterns and contourite drift occurrence to be When discussing modelled bottom currents, where possible we 88 23 demonstrated. In this paper, we present a census of the world’s provide the current name or alternatively specify the large-scale 89 24 known contourite features and use the database to assess the rela- water mass classification on the basis of previous work. Identify- 90 25 tionship between simulated bottom current activity and contourite ing regional-scale intermediate and deep-water masses requires a 91 26 distribution throughout the ocean. This work represents one part rigorous examination of the computed vertical stratification of the 92 27 of a growing effort to unite numerical methods with observations water column, and thus lies beyond the scope of the study. 93 28 from the geological record. 94 29 3. Results 95 30 2. Methods 96 31 3.1. Bottom current activity and global contourite distribution 97 32 2.1. Distribution of modern contourites 98 33 The most energetic bottom currents are simulated along the 99 34 An exhaustive review of the literature was conducted to assess western boundaries of ocean basins, near deep water creation sites, 100 35 and refine the known coverage of modern sediment drifts. Two and in areas that are tightly constricted by topography. Such re- 101 36 major existing databases were used as a basis for compiling the gions are associated with higher computed bottom current metrics 102 37 distribution of contourites used in this study.
Details
-
File Typepdf
-
Upload Time-
-
Content LanguagesEnglish
-
Upload UserAnonymous/Not logged-in
-
File Pages16 Page
-
File Size-