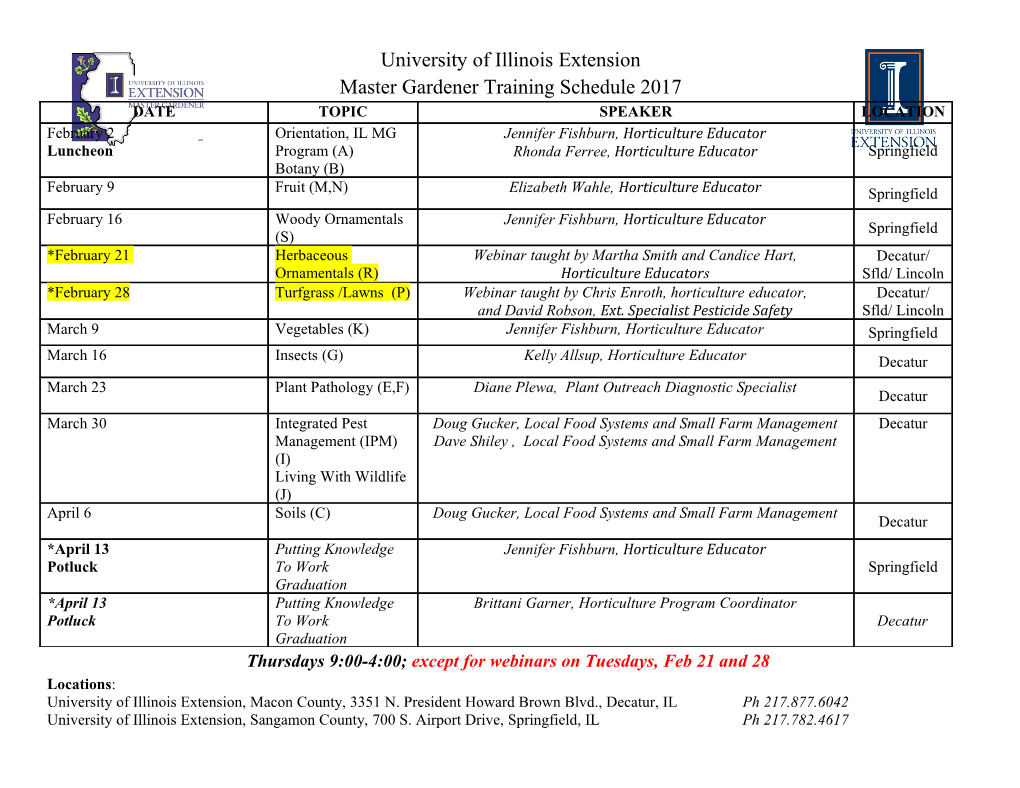
Resolving the Formation of Protogalaxies John H. Wise (KIPAC / Stanford) Thesis Advisor: Tom Abel Collaborators: Matt Turk (KIPAC), Greg Bryan (Columbia) 1 Outline ‣ Introduction to The Dark Ages, First Stars, and First Galaxies ‣ Observational and Theoretical Motivations ‣ Standard Galaxy Formation Models ‣ Molecular Hydrogen Cooling ‣ Primordial Star Formation and Feedback ‣ Effects on Protogalaxy Formation ‣ Physical and Computational Models 2 Early Structure Formation Illustration Credit: NASA and A. Feild (STScI) 3 CMB and The Dark Ages ‣ With the WMAP results, the conditions at this epoch are very well calibrated. Spergel et al. (2003, 2006) Credit: NASA/WMAP Science Team ‣ In CDM models, DM can clump on the free- streaming scale. ‣ However, baryons experience pressure forces and must overcome the cosmological Jeans mass before collapsing. ‣ Residual free electrons that exist after recombination aid the formation of molecular hydrogen. Saslaw & Zipoy (1967), Peebles & Dicke (1968), Tegmark et al. (1997) 4 The First Stars ‣ Various computational techniques have calculated and verified that the first stars are massive (30 - 300 M⊙) and isolated. Abel et al. (2002), Bromm et al. (2002), Yoshida et al. (2003) 6 50 ‣ L ~ 10 L⊙, ~10 ionizing photons / sec ‣ Lifetime ~ 3 Myr Schaerer (2002) ‣ H2 is the main coolant, which is easily dissociated by distant sources of radiation. Dekel & Rees (1987) ‣ Provide the first ionizing radiation and first metals to the Universe. Viz: Kähler, Wise, & Abel 5 The First Stars Heger et al. (2003) 6 The First Galaxies ‣ The transition from primordial stars to “normal” star formation is determined by the nature of the Bor stellar population. ne et al. (2001) ‣ Clustered and metal-enriched (Salpeter IMF) stellar population 4 ‣ Traditionally halos with Tvir > 10 K are considered (proto)galaxies, where gas can cool and collapse through hydrogen lines. Rees & Ostriker (1977), White & Rees (1978) Spitzer (1978) ‣ H2 cooling is enhanced by the free electrons created from this ionization in the first stars and galaxies. O’Shea et al. (2005) 7 Observational Motivations 9 ‣ z ~ 6 SMBHs have up to 10 M⊙ ‣ Gunn-Peterson troughs observed in z > 6 QSOs Becker et al. (2001), Fan et al. (2002) ‣ WMAP electron scattering optical depth indicates a reionization of z ~ 10 if a sudden reionization is assumed. Page et al. (2006) ‣ Halo and dwarf galaxy stellar metallicities Tolstoy et al. (2004), Qian & Wasserburg (2005) ‣ Lyman alpha metallicities Credit: SDSS Songaila & Cowie (1995), Songaila (2001) 8 Theoretical Motivations ‣ Hierarchical structure formation – the dynamics and history of smaller galaxies influences all later objects. Peebles & Dicke (1968), White & Rees (1978) ‣ Currently, observations only probe the most massive objects at z > 6. ‣ Protogalaxies are responsible for most of reionization in many semi- analytic models. Cen (2003), Ciardi et al. (2003), Ricotti & Ostriker (2003) ‣ How does the transition from W primordial to “normal” star echsler et al. (2002) formation occur? Metal mixing may play a large role in this determination. 9 Standard Galaxy Formation Model ‣ White & Rees (1978) set the stage for standard galaxy formation models by embedding galaxy formation within hierarchical structure formation. H + He ‣ Expanded by White & Frenk (1991) to include better calibrated star formation models and metallicity Barkana & Loeb (2001) effects. H2 ‣ DM and baryons are treated as solid body rotators. e.g. Loeb & Rasio (1994), Mo et al. (1998) ‣ Star formation in a rotationally supported disk. 10 Simulation Setup ‣ enzo – Adaptive Mesh Refinement ‣ Refine on dark matter and gas densities and resolve the Jeans length by at least 16 cells. ‣ 22 179 grids ‣ 74 000 000 (4203) unique cells ‣ Hydrogen and helium cooling only ‣ 1.5 comoving Mpc box ‣ Initial redshift = 120 11 Zoom by a factor 1012 Dynamic range of 1015 in length and 1025 in density, 41 levels of refinement 12 Turbulent Collapse within the Standard Galaxy Formation Model 7 ‣ Mass = 3.6 x 10 M⊙, Tvir = 9900 K, z = 16.8 Density ‣ There is no rotationally supported disk, but a thick, pressure supported disk with a radius of ~50 pc. 5 ‣ 10 M⊙ in the central parsec becomes gravitationally unstable and has a Mach number of 2-3. We expect a SMBH to form in this case. Loeb & Rasio (1994), Bromm & Loeb (2003), Spaans & Silk (2006) Begelman et al. (2006), Lodato & Natarajan (2006) ‣ No fragmentation down to sub-solar scales. 3 pc ‣ Central collapse occurs before any fragmentation in the disk. 13 Turbulent Collapse within the Standard Galaxy Formation Model v v ‣ Baryons undergo violent relaxationr r -30 -20 -10 0 10 20 30 -20 -10 0 10 20 30 similar to DM.0.1 0.1 z = z z = z Adiabatic / Particles c,H2 c,Lyα Adiabatic / Baryons 0.08 Cooling / Particles 0.08 ‣ Maxwellian (not a solid body Cooling / Baryons rotator!) velocity0.06 distribution 0.06 0.04 0.04 ‣ Angular momentum segregation. 0.02 0.02 Due to Rayleigh’tot s inviscid rotational tot 0 0 stability argument:z = z z = z 2 c,H2 c,Lyα M / 0.08dj 0.08 M / > 0 0.06dr 0.06 ‣ Only the lowest0.04 AM gas filters to the 0.04 central parsec.0.02 0.02 0 0 ‣ Afterwards, gravitational-30 -20 -10 bar0 10 20 30 -20 -10 0 10 20 30 v v instabilities. θ θ Shlosman et al. (1989, 1990), Begelman et al. (2006) 14 Radial profiles of the halo 8 ] 10 9500 7 (a) (c) solar 10 6 9000 10 5 10 8500 4 10 3 8000 10 2 10 7500 Temperature [K] 1 10 Enclosed Gas Mass [M 12 0 10 11.8 Myr 10 1.19 Myr 10 183 kyr –2.1 36.4 kyr 8 r 13.9 kyr 10 1.48 kyr ] -5 6 229 yr -3 10 z = 16.802317 [km/s] 4 s 10 , c n [cm 2 10 -10 r,gas v 0 10 (d) -2 (b) 10 -15 -4 -3 -2 -1 0 1 2 3 0 1 2 3 4 5 6 7 10 10 10 10 10 10 10 10 10 10 10 10 10 10 10 10 Radius [pc] Enclosed Gas Mass [Msolar] 15 Radial profiles of the inner parsec ] 10000 4 10 solar 9500 2 10 9000 0 10 8500 -2 10 8000 -4 Temperature [K] 10 7500 Enclosed Gas Mass [M -6 7000 1022 42.2yr (lvl 25) 10 9.46yr (lvl 27) 0 20 2.85yr (lvl 29) 10 0.88yr (lvl 31) 18 –2.1 62.5d (lvl 33) 10 r 26.9d (lvl 35) -5 ] 16 4.52d (lvl 37) -3 0.49d (lvl 39) 10 0 (lvl 41) 14 r,gas 10 -10 v n [cm 12 10 10 10 -15 8 10 6 -20 10 -8 -7 -6 -5 -4 -3 -2 -1 -6 -5 -4 -3 -2 -1 0 1 2 3 4 5 10 10 10 10 10 10 10 10 10 10 10 10 10 10 10 10 10 10 10 10 Radius [pc] Enclosed Gas Mass [Msolar] 16 Turbulent Collapse within the Standard Galaxy Formation Model ‣ Whether the central object be a starburst or SMBH, its feedback will have grand consequences on later star formation within the halo / disk. Density ‣ Ignoring H2 chemistry and primordial star formation made this simulation possible. ‣ This may never happen in nature, but it provides a good testbed for turbulent collapses and what the standard simulations of galaxy formation should find. ‣ What have we learned in this stage? 1.7 kpc The halo is turbulent and not rigidly rotating. It centrally collapses without fragmentation before disk formation. 17 Molecular Hydrogen Cooling ‣ H2 cooling is efficient to 300K at high densities. ‣ Easily dissociated by (Lyman-Werner) radiation between 11.2 – 13.6 eV. H + He Field et al. (1966), Stecher & Williams (1967) ‣ Soft UV backgrounds and nearby sources are important to quantify. H2 ‣ Primordial star formation is not halted Barkana & Loeb (2001) by this radiation but only delayed. ‣ The halo mass required to cool and collapse increases with the background intensity. Machacek et al. (2001), Wise & Abel (2005) 18 Molecular Hydrogen Cooling ‣ H2 cooling is efficient to 300K at high densities. 500 M⊙ 100 M⊙ ‣ Easily dissociated by (Lyman-Werner) H + He radiation between 11.2 – 13.6 eV. Field et al. (1966), Stecher & Williams (1967) ‣ Soft UV backgrounds and nearby sources are important to quantify. H2 ‣ Primordial star formation is not halted by this radiation but only delayed. Wise & Abel (2005) ‣ The halo mass required to cool and collapse increases with the background intensity. Machacek et al. (2001), Wise & Abel (2005) Redshift 19 H2 Cooling Simulations J = 10-22 J = 10-21 No UV Background UV Background UV Background No residual e– No residual e– Hydrogen & Helium J = 10-20 Only No UV Background UV Background 20 H2 Cooling Simulations Density 1.7 kpc z = 31.1 z = 28.3 z = 24.4 z = 23.4 z = 21.1 z = 16.8 21 H2 Cooling Simulations Temperature 1.7 kpc z = 31.1 z = 28.3 z = 24.4 z = 23.4 z = 21.1 z = 16.8 22 H2 Cooling Simulations 8 10 ] 7 sun 10 6 10 H2 Halo Mass [M H2LW22 H2LW21 noe-H2 noe-H2LW20 H+He 5 10 2.5 3 3.5 4 30 25 20 1510 10 10 10 Redshift Central Temperature [K] 23 H2 Cooling Simulations ‣ Even in the worst case scenario with no residual electrons and a soft UV background of J = 10-20, we see halos cooling and collapsing before virial temperatures reach 104 K. ‣ Critical minimum masses are ~3x (~5x with no UV background and no residual electrons) smaller than previously thought. ‣ Due to the exponential nature of Press-Schechter formalism, this results in an order of magnitude increase of protogalaxies.
Details
-
File Typepdf
-
Upload Time-
-
Content LanguagesEnglish
-
Upload UserAnonymous/Not logged-in
-
File Pages43 Page
-
File Size-