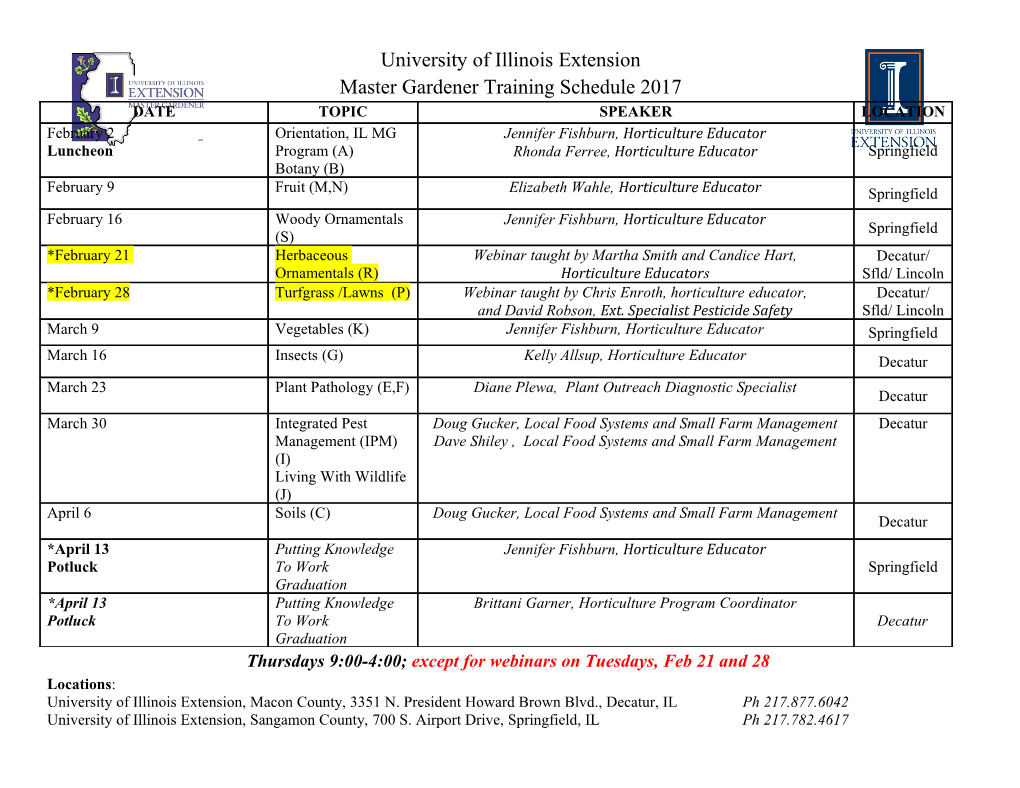
University of Central Florida STARS Electronic Theses and Dissertations, 2020- 2021 On the Interaction of Creep and High-Cycle Fatigue in CM 247 LC Thomas Bouchenot University of Central Florida Part of the Mechanical Engineering Commons Find similar works at: https://stars.library.ucf.edu/etd2020 University of Central Florida Libraries http://library.ucf.edu This Doctoral Dissertation (Open Access) is brought to you for free and open access by STARS. It has been accepted for inclusion in Electronic Theses and Dissertations, 2020- by an authorized administrator of STARS. For more information, please contact [email protected]. STARS Citation Bouchenot, Thomas, "On the Interaction of Creep and High-Cycle Fatigue in CM 247 LC" (2021). Electronic Theses and Dissertations, 2020-. 475. https://stars.library.ucf.edu/etd2020/475 ON THE INTERACTION OF CREEP AND HIGH-CYCLE FATIGUE IN CM 247 LC by THOMAS S. BOUCHENOT B.S. University of Central Florida, 2013 M.S. University of Central Florida, 2015 A dissertation submitted in partial fulfillment of the requirements for the degree of Doctor of Philosophy in the Department of Mechanical and Aerospace Engineering in the College of Engineering and Computer Science at the University of Central Florida Orlando, Florida Spring Term 2021 Major Professor: Ali P. Gordon © 2021 Thomas S. Bouchenot ii ABSTRACT Industrial gas turbines constitute a considerable portion of global power generation, from large engines for centralized grids, to smaller frames for decentralized demand. Power output, efficiency, and reliability are chief criteria regarding the development of next-generation turbines, whose components are expected to operate in ever harsher environments. Turbine blades are subjected to high temperatures, an array of mechanical loads, and dynamic fluid fields, all contained within a non-isothermal profile, making creep and high-cycle fatigue critical aspects of turbine blade design. Current analysis tools and methods have resulted in the development of many successful components; however, improved life prediction models with even greater accuracy are needed to maintain today’s product reliability under the extreme environments associated with next-generation operating conditions. The combination of creep and high-cycle fatigue produces a synergistic interaction effect, whose explicit consequence to turbine life remains unaccounted for by current, decoupled life prediction models, which traditionally incorporated such interaction effects into design safety factors. The continued development of high fidelity analytical tools, unique cooling technologies, and advanced materials has propelled turbine design to higher efficiencies and tighter margins. Improved lifing models capable of capturing these effects are now needed in order to maintain current reliability standards in these ever-expanding, future operating conditions. Combined creep and high-cycle fatigue has been the subject of very little research; however, sufficient knowledge is available in literature to guide the formation of a comprehensive study. This research study identifies the life-limiting aspect of a combined high-cycle fatigue and creep response in conventionally cast Ni-base superalloy CM 247 LC, and captures the interaction of the two loads in two novel candidate life prediction frameworks. The frameworks iii are created from a comprehensive collection of experimental data obtained using a conventional high-cycle fatigue test method and an unconventional two-part test method, where test specimens pre-deformed to a prescribed creep strain are fatigue loaded at an elevated temperature and high frequency until failure. A variety of temperatures, creep strains, and fatigue loading conditions are be explored to ensure that the resulting model is applicable to the myriad of potential turbine blade operating conditions. Additional tests are also be carried out to determine the contribution of high temperature aging on the combined failure and to evaluate the effect of material grain size. Rigorous metallographic assessments accompanying each test are leveraged to create the microstructurally-informed combined life prediction frameworks. iv To my wife and parents v ACKNOWLEDGEMENTS The assistance and support of many co-workers, friends, and family were critical to the completion of this disseration. Several individuals deserve special acknowledgement. My success as an engineer and researcher must largely be credited to the mentorship of Dr. Ali P. Gordon. His guidance, hard work and dedication as an advisor, teacher, and role model has been the foundation of my academic and professional career. I cannot thank him enough. I would like to thank Dr. Seetha Raghavan, Dr. Kevin Mackie, Dr. Ranajay Ghosh, and Dr. Felipe Viana for serving as my disseration committee members. I would also like to thank Siemens Energy for the opportunity and funding to conduct the research utilized in this dissertation. In particular, I would like to thank my manager at Siemens, Mandy Goltsch, and the lifing methods manager at Siemens, Sachin Shinde. I would not have been able to complete my dissertation without their invaluable support. Ronnie Bladh will always have my gratitude and respect. His mentorship in the field of aeromechanics and applied gas tubine high-cycle fatigue life prediction have been a great influence and learning experience. I also want to think Kirtan Patel for always supplying his expert recommendations on material characterization. My discussions with Allister James and Phillip Draa have also been a great help with regards to understanding superalloy microstructure. I am also thankful for the support of my other past and present Siemens colleagues, including Zach Dyer and Kyle Smith. I would also like to express my deepest thanks to the technicians and engineers at Siemens Casselberry lab for their significant contributions to the completion of this dissertation. I am especially grateful for the assistance of Dillon Ryan and David Kosich for conducting many vi of the experiments used in this study, as well as the help and expertise of Tom Brumley, without whom the post-test fracture analysis could not have been completed. I am also thankful for the help of Brooke Sarley for keeping the project on schedule by interfacing with the test vendors and coordinating the specimen preparation. I would also like to thank all the past and present friends and colleagues in the Mechanics of Materials Research Group (MOMRG) at the University of Central Florida (UCF). I have learned a substantial amount from them over the years as both an undergraduate and graduate student. Collaborations and discussions with Justin Karl, Scott Keller, Steven Kraft, Calvin Stewart, Kevin Smith, Jonathan Torres, Nathan O’Nora, Sanna Saddiqui, Bassem Felemban, Firat Irmak, and the numerous other lab members have helped me grow as a engineer and researcher. I could not have achieved so much without the support of my family. The care and encourgement of my parents and brother have been driving force in my education and development. I would never have finished my doctorate without the love and compassion of my wife, Jenny. She has always been there to cheer me on when I was starting to lose sight of the finish line, and always convinced me to take a break whenever I would begin to wear out. Knowing that she will always be there for me has made a tremendous difference on my graduate career and my life as a whole. vii TABLE OF CONTENTS LIST OF FIGURES ...................................................................................................................... xii LIST OF TABLES ..................................................................................................................... xxiii LIST OF NOMENCLATURE .................................................................................................... xxv CHAPTER 1 INTRODUCTION .................................................................................................... 1 CHAPTER 2 MATERIAL ............................................................................................................ 10 2.1 Processing ........................................................................................................................... 10 2.2 Microstructure ..................................................................................................................... 12 2.2.1 Composition ................................................................................................................. 12 2.2.2 Grain Details ................................................................................................................ 14 2.3 Elastic and Tensile Response .............................................................................................. 16 CHAPTER 3 LITERATURE REVIEW ....................................................................................... 22 3.1 High-Cycle Fatigue ............................................................................................................. 22 3.1.1 The HCF Load Cycle ................................................................................................... 24 3.1.2 HCF Testing ................................................................................................................. 26 3.1.3 Presenting HCF Results ............................................................................................... 27 3.1.4 Classical HCF Failure Criteria ....................................................................................
Details
-
File Typepdf
-
Upload Time-
-
Content LanguagesEnglish
-
Upload UserAnonymous/Not logged-in
-
File Pages386 Page
-
File Size-