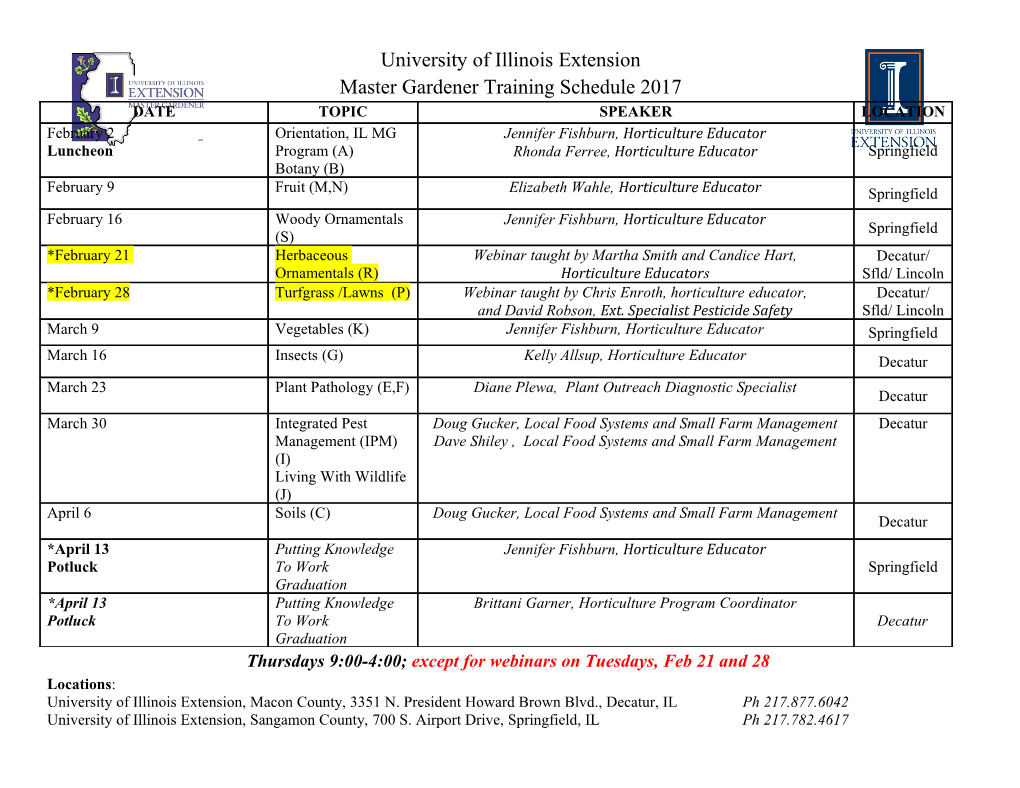
PHYSICAL REVIEW D 102, 094014 (2020) Covariantizing phase space † Andrew J. Larkoski 1,* and Tom Melia2, 1Physics Department, Reed College, Portland, Oregon 97202, USA 2Kavli Institute for the Physics and Mathematics of the Universe (WPI), UTIAS, The University of Tokyo, Kashiwa, Chiba 277-8583, Japan (Received 21 August 2020; accepted 21 October 2020; published 16 November 2020) We covariantize calculations over the manifold of phase space, establishing Stokes’ theorem for differential cross sections and providing new definitions of familiar observable properties like infrared and collinear safety. Through the introduction of explicit coordinates and a metric we show phase space is isomorphic to the product space of a simplex and a hypersphere, and we identify geometric phenomena that occur when its dimensions are large. These results have implications for fixed-order subtraction schemes, machine learning in particle physics and high-multiplicity heavy ion collisions. DOI: 10.1103/PhysRevD.102.094014 Relativistic N-body phase space is the manifold on divergences from unresolved collinear or very low-energy which essentially all calculations in a perturbative quantum particles exactly cancel [1–4]. Making a mathematically field theory take place. S-matrix elements are functions that rigorous statement of precisely how IRC safety constrains live on the phase space manifold and can exhibit diver- observables is known to have problems [5]. Much of the gences on degenerate subspaces. Experiments extrapolate challenge is related to the technical fact that real and virtual smooth probability distributions from discrete, finite data of divergences only need to strictly cancel in the exact soft particles’ momenta. The first step of any Monte Carlo and/or collinear limit, but a lack of a smoothness can render generator for fixed-order calculation or parton shower perturbative predictions pathological slightly away from simulation involves the sampling of points on phase space. these limits. Nevertheless, some progress has been made by In each of these cases, phase space itself is often treated as either restricting to a smaller class of observables or the background on which the calculations take place, with exploiting smoothness properties of the space of collections little focus on its intrinsic geometry. of particles equipped with a metric [6]. We make a In this paper, we present a covariant description of phase conjecture for a definition of IRC safety based on the space and elucidate some of its novel geometric properties. validity of Stokes’ theorem. Our aim is to demonstrate that a deeper understanding of Establishing the phase space manifold is also important phase space can enable the identification of restrictions on for applications of machine learning in particle physics differentiable functions that can live on it, bring new [7–11]. The space in which the data input to the machine interpretations of fundamental quantities like differential lives can be used to optimize its architecture, as exploited in cross sections, and broaden the questions that can be asked convolutional or recurrent neural networks, and a neural of particle physics data. network equivariant under the Lorentz group has recently We establish the application of Stokes’ theorem to been constructed [12]. Recent work has shown that phase differential cross sections, viewing observables as provid- space in four dimensions is a Stiefel manifold modulo the ing foliations of the phase space manifold. This sheds new little group [13,14], see also [15]. Optimization and machine light on the criteria of infrared and collinear (IRC) safety learning on Stiefel manifolds is well explored in other fields, and additivity of an observable. These properties play a particularly for pattern recognition, e.g., [16–22]. special role in massless gauge theories in four dimensions We introduce explicit global coordinates that enable the —only through the calculation of IRC safe observables do construction of a metric and other quantities on phase space, providing essential input and new ways of organ- *[email protected] izing data to machine learning applications. Another † [email protected] promising application of explicit metrics on phase space is to provide natural distance measures that can act as Published by the American Physical Society under the terms of regularization observables; such observables are employed the Creative Commons Attribution 4.0 International license. in techniques [23–36] to isolate soft and collinear diver- Further distribution of this work must maintain attribution to the author(s) and the published article’s title, journal citation, gences in modern efforts to push calculations in perturba- and DOI. Funded by SCOAP3. tion theory to high orders in QCD. 2470-0010=2020=102(9)=094014(8) 094014-1 Published by the American Physical Society ANDREW J. LARKOSKI and TOM MELIA PHYS. REV. D 102, 094014 (2020) Z pffiffiffi We also establish geometric phenomena that occur on the D−1 ˜ ˜ i FðOÞ¼ d x g NiV ; ð3Þ phase space manifold when particle multiplicity is large Σ and derive new geometric test statistics in this limit. Specifically, the “curse of dimensionality” forces the phase where we define the vector space volume to concentrate at the boundaries of phase — ij∇ space we show in our explicit coordinate system that this i g jhðxÞ V ¼ MðxÞ kl : ð4Þ implies light cone momenta of particles are squeezed to the g ∇khðxÞ∇lhðxÞ boundaries of a simplex. Such high-dimensional geometry has a natural application in heavy ion physics, where This vector Vi describes a flow in phase space along the multiplicities are large. gradient of observable O, Let Π denote the phase space manifold of dimension D. Anticipating a covariant description, we introduce local dxi M x Vi: coordinates xi and metric g on Π and write the phase space ð Þ O ¼ ð5Þ pffiffiffi d measure in terms of the metric as dDx g. We consider an integral of a function MðxÞ over phase space, restricted to a We will show shortly that such an interpretation is useful in hypersurface Σ, defined by hðxÞ¼O, via a δ-function, classifying properties of observables. ’ Z Equation (3) is now in a form where Stokes theorem, in pffiffiffi covariant form, can be readily applied. Consider a volume FðOÞ¼ dDx gMðxÞδðO − hðxÞÞ: ð1Þ Ω Π ∂Ω Π in with closed boundary defined by two hyper- surfaces Σ1 and Σ2, corresponding to two values of the If MðxÞ is a squared S-matrix element, then FðOÞ is observable h ¼ O1 and h ¼ O2 [see Fig. 1(a)], then we interpreted as a differential cross section for O, as defined have, by Stokes, by the function hðxÞ on phase space. This notation makes Z Z the possible multiple real and virtual contributions to the i i FðO1Þ − FðO2Þ¼ dσiV − dσiV matrix element implicit. This integral form also describes ZΣ1 Σ2 observables defined by smooth weights on phase space, ffiffiffi D p i like the energy-energy correlation function [37]. At this ¼ d x gDiV ; ð6Þ Ω point we also notep thatffiffiffiffiffiffiffiffiffi as MðxÞ ≥ 0, it is possible to 2 interpret the quantity gM as a volume form and a metric where the definition of the surface element dσi can be be associated with the dynamical theory; we return to this inferred from Eq. (3), and the covariant divergence is interesting possibility below. given by The δ-function has the effect of turning Eq. (1) into an i Σ i ∇ i Γj i integral of a vector V over the hypersurface . To see this, DiV ¼ iV þ ijV ; ð7Þ we change to coordinates ðh; x˜aÞ, defining the induced metric on Σ, with the contracted Christoffel symbols pffiffiffi ∂xi ∂xj Γj ∇ log g: 8 g˜ ¼ g : ð2Þ ij ¼ i ð Þ ab ij ∂x˜a ∂x˜b In general, a closed boundary on phase space could involve ˜ hh hh Using the identity detðgijÞ¼detðgabÞ=g , where g ¼ (subsets of) the boundary of phase space—see Fig. 1(b) for ij g ∇ih∇jh, introducing the normal covector to the hyper- an illustration. kl 1=2 surface, Ni ¼ð∇ihÞ=ðg ∇kh∇lhÞ , and performing the As an illustrative example, we consider the foliation of now trivial integral over the δ-function in these coordinates, phase space by the C-parameter [38–40] in eþe− → qqg¯ 2 it follows that Eq. (1) becomes events. Defining the variables xi ¼ 2pi · Q=Q , where pi is FIG. 1. (a) Stokes’ theorem applied to a subvolume Ω of Π bounded by two hypersurfaces Σ1 and Σ2 that are foliations defined by observable values O1 and O2. (b) The case for which the boundary of subvolume Ω includes part of the boundary ∂Π of the full space. (c) C-parameter foliation of three-body phase space in the xi coordinates introduced in the text. 094014-2 COVARIANTIZING PHASE SPACE PHYS. REV. D 102, 094014 (2020) the momentum of particle i ¼ 1, 2, 3 and Q is the total and collinear safe if the quantity FðOÞ in Eq. (3) is momentum vector of the collision, we choose three-body calculable on all hypersurfaces defined by hðxÞ. It follows phase space coordinates (x1, x2). The C-parameter sets that the lhs of Eq. (6) is calculable. The textbook statement of conditions for which Stokes’ 1 − 1 − 1 − Ω 6 ð x1Þð x2Þð x3Þ theorem holds is that the manifold is smooth, and that the hðxÞ¼ ; ð9Þ − 1 ∂Ω ðx1x2x3Þ D form that is integrated over the boundary is smooth and has compact support on Ω. We make the with x3 ¼ 2 − x1 − x2, and we plot its contours in Fig. 1(c). conjecture that the same conditions for Stokes’ theorem to In these coordinates the manifold is flat, gij ¼ δij, the hold in Eq. (6) are those that dictate IRC safety, namely that i i gradient is ∇ ¼ð∂=∂x1; ∂=∂x2Þ, and the vector field V in the D − 1 form that is integrated over the hypersurface Σ in Eq.
Details
-
File Typepdf
-
Upload Time-
-
Content LanguagesEnglish
-
Upload UserAnonymous/Not logged-in
-
File Pages8 Page
-
File Size-