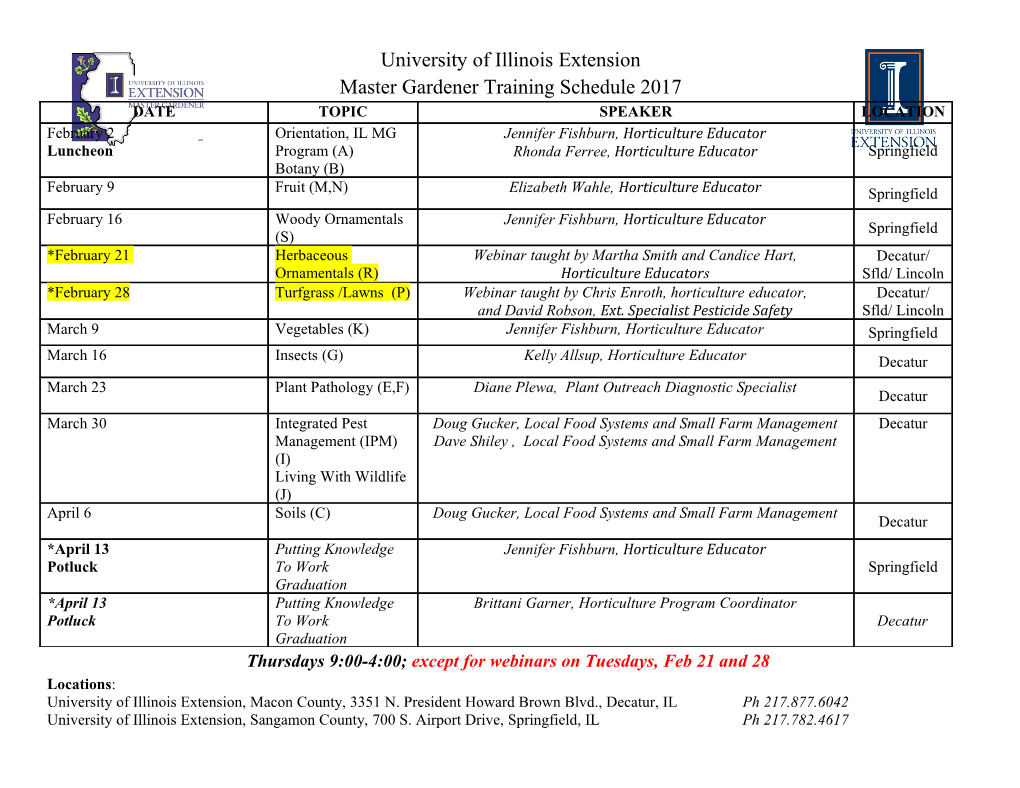
Earth and Planetary Science Letters 189 (2001) 75^84 www.elsevier.com/locate/epsl Degree-1 mantle convection and the crustal dichotomy on Mars Shijie Zhong *, Maria T. Zuber Department of Earth, Atmospheric and Planetary Sciences, Massachusetts Institute of Technology, Cambridge, MA 02139-4307, USA Received 18 October 2000; received in revised form 26 March 2001; accepted 11 April 2001 Abstract The surface of Mars consists of an old, heavily cratered and elevated southern hemisphere and younger, resurfaced and depressed northern hemisphere, a feature often termed the crustal dichotomy. The global crustal structure [Zuber et al., 2000] revealed by topography and gravity data from the Mars Global Surveyor spacecraft, and the possible late formation of the boundary zone between the hemispheres [McGill and Dimitriou, 1990], have been proposed to indicate an endogenic origin for the dichotomy. However, degree-1 mantle convection that is required for any endogenic process to be viable cannot be produced with conventional mantle convection models [Schubert et al., 1990]. We have studied the role of radially stratified viscosity on mantle deformation by using Rayleigh^Taylor instability analyses in a spherical shell geometry. Our analyses reveal that when mantle viscosity is stratified with a weak asthenosphere, deformation at long wavelengths is more efficient than that at short wavelengths. The weaker the asthenosphere, the longer the wavelength at which the deformation is the most efficient. A thicker asthenosphere also favors the deformation at long wavelengths. Both the Rayleigh^Taylor instability analyses and numerical modeling of mantle convection show that degree-1 convection can be produced within the Martian mantle provided that the mantle had a weak asthenosphere (V500 km thick and V102 times weaker than the underlying mantle) early in planetary history. The degree-1 convection causes preferential heating of one hemisphere that may explain the primary features associated with the dichotomy in crustal structure. ß 2001 Elsevier Science B.V. All rights reserved. Keywords: mantle; convection; Mars; mantle rheology; crust 1. Introduction cesses include single [7] and multiple [8] hemi- spheric-scale impacts. An impact-related mecha- Since the Martian crustal dichotomy was ¢rst nism implies that the dichotomy is a primordial identi¢ed by the Mariner 9 spacecraft [4], both feature [2] formed when large impactors in planet- endogenic [5,6] and exogenic [7,8] processes have crossing orbits were still present in the post-accre- been proposed to explain its origin. Exogenic pro- tional solar system. However, an impact origin may be di¤cult to reconcile with recent Mars Global Surveyor (MGS) geophysical observations that indicate pole-to-pole gradual variations in to- * Corresponding author. Present address: Department of Physics, University of Colorado at Boulder, Boulder, CO pography [9] (Fig. 1a) and crustal thickness [1] 80309, USA. Tel.: +1-303-735-5095; Fax: +1-303-492-7935. and a mismatch between the surfacial expression 0012-821X / 01 / $ ^ see front matter ß 2001 Elsevier Science B.V. All rights reserved. PII: S0012-821X(01)00345-4 EPSL 5855 5-6-01 Cyaan Magenta Geel Zwart 76 S. Zhong, M.T. Zuber / Earth and Planetary Science Letters 189 (2001) 75^84 Fig. 1. (a) Molleweide projection of the topography of Mars updated from Smith et al. [9]. The geological expression of the di- chotomy boundary occurs approximately at the green to blue transition. In the map zero longitude runs down the center of the ¢gure. (b) Pole-to-pole slice of topography and crustal thickness [1] along 0³ longitude illustrating planet-scale (degree-1) struc- ture. The mean crustal thickness in the north is V40 km (left to center) and increases to V75 km at high southern latitudes (right). of the dichotomy and the crustal structure (Fig. the observed geological and geophysical con- 1b) [1]. In addition, geological mapping suggests straints. An early study suggested on the basis that at least part of the boundary formed later in of qualitative arguments that the thinner crust in Martian history (Late Noachian or Early Hesper- the northern hemisphere could be a consequence ian) [2,10] than would be expected if produced by of sub-crustal erosion caused by unspeci¢ed de- a mega-impact or impacts. gree-1 mantle convection [5]. More recently, Sleep Endogenic processes could conceivably satisfy [6] proposed that the dichotomy boundary may EPSL 5855 5-6-01 Cyaan Magenta Geel Zwart S. Zhong, M.T. Zuber / Earth and Planetary Science Letters 189 (2001) 75^84 77 represent relic boundaries associated with plate to degree-1 convection that can explain the gen- tectonics in the northern hemisphere. The con¢r- eral characteristics of the global topography and mation of the impact origin of the 1500-km diam- crustal structure. eter Utopia structure [2] in the northern hemi- sphere from the MGS topography [9] and gravity [11] observations suggest that if the hemi- 2. Physical models spheric di¡erence was a consequence of plate tec- tonics, it must have originated early in solar sys- To study the generation of degree-1 structure, tem history. The recent discovery of zones of we have employed two di¡erent approaches: Ray- alternating magnetization in the southern hemi- leigh^Taylor instability analyses and ¢nite ele- sphere [12] has been interpreted as evidence of ment models of thermal convection. We describe spreading of the early Martian crust [13]. How- our model approaches in this section. ever, the crust in this region does not have a structure that is obviously suggestive of plate tec- 2.1. A Rayleigh^Taylor instability analysis tonics [1] and other tectonic processes, such as magmatic intrusion [14], may alternatively explain A Rayleigh^Taylor instability analysis may the magnetic lineations. On the other hand, if the provide important insights into the conditions average crustal thickness is V50 km [1], it is un- under which degree-1 convection may occur [22]. clear whether mantle convection could result in Our analytic methods are based on a recently de- subduction of such a thick crust, given that the veloped model for viscoelastic stress relaxation in basalt^eclogite phase transition, which aids the a spherical shell geometry [23]. For our Rayleigh^ subduction, does not occur until a depth of Taylor instability analysis, the mantle is consid- V200 km. ered to be purely viscous. In addition, the mantle In order for an endogenic process (such as but is assumed incompressible with two layers of dif- not restricted to plate tectonics) to be a viable ferent viscosity and density. The dynamics is de- mechanism to explain a global feature like the termined by the equations of continuity and mo- crustal dichotomy, planet-scale (degree-1) mantle tion: convection is necessary [3]. However, mantle con- ! vection models with either isoviscous [3] or tem- 9W u 0 1 perature-dependent viscosity [15,16] structure do ! T ! ! not produce degree-1 convection. Core formation 39P 9 W R 9 u 9 u 3bger 0 2 may result in degree-1 convection [3,17], but if this process produced the dichotomy, then evi- where !u is the velocity, P is the pressure, R is the ! dence for early core formation on Mars [18] viscosity, g is the gravitational acceleration, e r is would also imply a primordial origin for the di- the unit vector in vertical direction, and b is the chotomy. Such a scenario would require addition- density. The surface and core^mantle boundary al mechanisms to explain both the resurfacing of (CMB) are subjected to stress-free boundary con- the northern hemisphere [19] and the relatively ditions. Similar analyses in a Cartesian geometry young age for at least part of the dichotomy are used in studying the structure of salt domes boundary [2,11]. The dynamics of solid-state [24,25]. The density interface is at r = rd . For the phase changes may produce a single thermal up- upper layer with r s rd , b = bu and R = Ru. For 3 welling plume (i.e., approximately degree-1 fea- r 6 rd , b = bl (i.e., 3400 kg m in Table 1) and ture), which has been used to explain the Tharsis R = Rl.Ifbl 6 bu, then the two-layer system is un- volcano-tectonic province on Mars [20,21]. How- stable. If a perturbation at a wavelength or spher- ever, a single upwelling plume can only form after ical harmonic degree is introduced to the topog- 2U109 years [20,21], which may be too late to raphy of the density interface, the topography will explain the formation of dichotomy [2]. In this grow with time as the instability develops. For study we explore endogenic processes that lead our models with three deformable density interfa- EPSL 5855 5-6-01 Cyaan Magenta Geel Zwart 78 S. Zhong, M.T. Zuber / Earth and Planetary Science Letters 189 (2001) 75^84 ces including the surface and the CMB, the time Table 1 evolution of the interfacial topography H(l,t) Model parameters at harmonic degree l and at r = rd is controlled Parameter Value 6 by the sum of three exponential functions with Mars radius R0 3.4U10 m 6 di¡erent characteristic time dli (i = 1, 2 and 3) Radius of the CMB 1.65U10 m [23] Density of the mantle 3400 kg m33 a Heat production rate Hb(t) X3 Uranium concentration 25.7 ppb H l; t hliexp t=d li 3 Thermal expansivity 3U1035 K31 i1 Thermal di¡usivity 1036 m2 s31 Thermal conductivity 3.0 W K31 m31 where h is the eigenfunction. The characteristic Surface gravitational acceleration 3.7 m s32 li 31 time d depends on the density and viscosity struc- Adiabatic temperature gradient 0.013 K MPa li Temperature at the CMBb 1950 K tures of the two-layer model [23].
Details
-
File Typepdf
-
Upload Time-
-
Content LanguagesEnglish
-
Upload UserAnonymous/Not logged-in
-
File Pages10 Page
-
File Size-