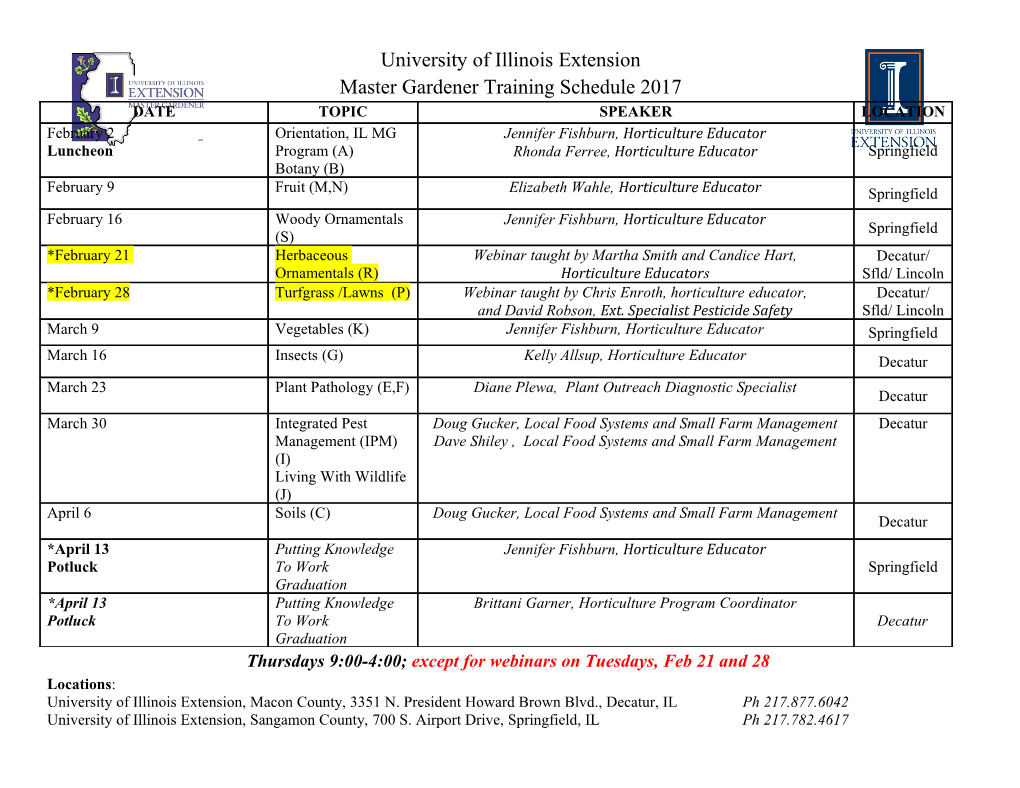
REVIEWS OF MODERN PHYSICS, VOLUME 75, APRIL 2003 Neutrino masses and mixing: evidence and implications M. C. Gonzalez-Garcia Theory Division, CERN, CH-1211, Geneva 23, Switzerland; Instituto de Fı´sica Corpuscular, Universitat de Vale`ncia–C.S.I.C, Edificio Institutos de Paterna, Apt. 22085, 46071 Vale`ncia, Spain; C.N. Yang Institute for Theoretical Physics, State University of New York at Stony Brook, Stony Brook, New York 11794-3840 Yosef Nir Department of Particle Physics, Weizmann Institute of Science, Rehovot 76100, Israel (Published 26 March 2003) Measurements of various features of the fluxes of atmospheric and solar neutrinos have provided evidence for neutrino oscillations and therefore for neutrino masses and mixing. The authors review the phenomenology of neutrino oscillations in vacuum and in matter. They present the existing evidence from solar and atmospheric neutrinos as well as the results from laboratory searches, including the final status of the Liquid Scintillator Neutrino Detector (LSND) experiment. The theoretical inputs that are used to interpret the experimental results are described in terms of neutrino oscillations. The allowed ranges for the mass and mixing parameters are derived in two frameworks: First, each set of observations is analyzed separately in a two-neutrino framework; Second, the data from solar and atmospheric neutrinos are analyzed in a three-active-neutrino framework. The theoretical implications of these results are then discussed, including the existence of new physics, the estimate of the scale of this new physics, and the lessons for grand unified theories, for models of extra dimensions and singlet fermions in the bulk, and for flavor models. CONTENTS 4. Global analysis 368 V. Atmospheric Neutrinos 369 A. Atmospheric neutrino fluxes 371 I. Introduction 346 1. Cosmic-ray spectrum 372 II. The Standard Model and Neutrino Masses 347 2. Geomagnetic effects 372 A. The standard model implies mϭ0 348 3. The neutrino yield 373 B. Extensions of the standard model allow mÞ0 348 4. The neutrino fluxes 373 C. Dirac and Majorana neutrino mass terms 349 B. Interaction cross sections 374 D. Lepton mixing 350 C. Two-neutrino oscillation analysis 375 III. Neutrino Oscillations 351 1. Predicted number of events 375 A. Neutrino oscillations in vacuum 351 B. Neutrinos in matter: Effective potentials 352 2. Conversion probabilities 376 C. Evolution equation in matter: Effective mass 3. Statistical analysis 376 ! and mixing 354 4. e 377 ! ! D. Adiabatic versus nonadiabatic transitions 355 5. and s 378 E. Propagation in the sun: Mikheyev-Smirnov- VI. Laboratory Experiments 379 Wolfenstein effect 357 A. Short-baseline experiments at accelerators 379 IV. Solar Neutrinos 358 B. LSND and KARMEN 379 A. Solar neutrino experiments 360 C. Disappearance experiments at reactors 381 1. Chlorine experiment: Homestake 360 D. Long-baseline experiments at accelerators 382 2. Gallium experiments: SAGE and E. Direct determination of neutrino masses 382 GALLEX/GNO 360 VII. Three-Neutrino Mixing 383 3. Water Cerenkov experiments: Kamiokande A. Probabilities 384 and SuperKamiokande 360 B. Allowed masses and mixing 386 4. SNO 361 VIII. Implications of the Neutrino Mass Scale and Flavor 5. Future: Borexino and low-energy Structure 387 experiments 362 A. New physics 387 B. The solar neutrino problem 363 B. The scale of new physics 388 C. Solar neutrino oscillation probabilities 364 C. Implications for flavor physics 388 1. Quasivacuum oscillations and the dark side 364 1. The flavor parameters 388 2. Evolution in the Earth 365 2. Special features of the neutrino flavor D. Two-neutrino oscillation analysis 366 parameters 389 1. Predictions 366 3. Large mixing and strong hierarchy 390 2. Analysis of total event rates: allowed IX. Implications for Models of New Physics 393 masses and mixing 367 A. Grand unified theories 393 3. Day-night spectra: Excluded masses and B. Extra dimensions 393 mixing 368 1. Coupling to bulk fermions 394 0034-6861/2003/75(2)/345(58)/$35.00 345 ©2003 The American Physical Society 346 M. C. Gonzalez-Garcia and Yosef Nir: Neutrino masses and mixing 2. Lepton number breaking on a distant brane 395 into a muon and a neutrino. The material in the dump 3. The warp factor in the Randall-Sundrum will eventually absorb the muons, photons, and any scenario 395 other charged particles, so that only neutrinos exit at the X. Conclusions 395 Note added 396 opposite end, forming an intense and controlled beam. KamLAND results 396 Neutrinos are also produced in natural sources. From Consequences for solar neutrinos 397 the 1960s on, neutrinos produced in the sun and in the Effect on three-neutrino mixing scenarios 398 atmosphere have been observed. As we shall see in this Acknowledgments 398 review, these observations play an important role in ex- References 398 panding our understanding of the detailed features of neutrinos. In 1987, neutrinos from a supernova in the I. INTRODUCTION Large Magellanic Cloud were also detected. The properties of the neutrino and in particular the In 1930 Wolfgang Pauli postulated the existence of the question of its mass have intrigued physicists ever since neutrino in order to reconcile data on the radioactive it was proposed (Kayser, Gibrat-Debu, and Perrier, decay of nuclei with energy conservation. In radioactive 1989; Ramond, 1999). In the laboratory neutrino masses decays, nuclei of atoms mutate into different nuclei have been searched for in two types of experiments when neutrons are transformed into slightly lighter pro- (Boehm and Vogel, 1987): (i) direct kinematic searches tons with the emission of electrons: of neutrino mass, of which the most sensitive is the study neutron!protonϩelectronϩantineutrino. (1) of tritium beta decay, and (ii) neutrinoless double- de- cay experiments. Experiments have achieved higher and Without the neutrino, energy conservation would re- higher precision, reaching upper limits for the electron quire that the electron and proton share the neutron’s Ϫ neutrino mass of 10 9 of the proton mass, rather than energy. Each electron would therefore be produced with the 10Ϫ2 originally obtained by Pauli. This raised the a fixed energy, while experiments indicated conclusively that electrons were not monoenergetic but were ob- question of whether neutrinos are truly massless like photons. served with a range of energies. This energy range cor- Ϫ9 responded exactly to the many ways the three particles Can one go further below the eV scale (that is, 10 of in the final state of the reaction above could share en- the proton mass) in the search for neutrino masses? This ergy while satisfying the conservation law. The postu- is a very difficult task in direct measurements. In 1957, lated neutrino had no electric charge and, for all practi- however, Bruno Pontecorvo realized that the existence cal purposes, did not interact with matter; it just served of neutrino masses implied the possibility of neutrino as an agent to balance energy and momentum in the oscillations. This phenomenon is similar to what hap- above reaction. In fact, Pauli pointed out that for the pens in the quark sector, where neutral kaons oscillate. neutrino to do the job, it had to weigh less than one Flavor oscillations of neutrinos have been searched for percent of the proton mass, thus establishing the first using either neutrino beams from reactors or accelera- limit on neutrino mass. tors, or natural neutrinos generated at astrophysical Observing neutrinos is straightforward—in principle. sources (the Sun giving the largest flux) or in the atmo- Pauli had to wait a quarter of a century before Fred sphere (as the by-products of cosmic-ray collisions). The Reines and Clyde Cowan, Jr. observed neutrinos pro- longer the distance that the neutrinos travel from their duced by a nuclear reactor. In the presence of protons, production point to the detector, the smaller the masses neutrinos occasionally initiate the inverse reaction of ra- that can be signalled by their oscillation. Indeed, the dioactive decay: solar neutrinos allow us to search for masses that are as Ϫ Ϫ ¯ϩp!nϩeϩ. (2) small as 10 5 eV, that is, 10 14 of the proton mass! In recent years, experiments studying natural neutrino Experimentally, one exposes a material rich in protons fluxes have provided us with the strongest evidence of to a neutrino beam and simply looks for the coincident neutrino masses and mixing. Experiments that measure appearance of an electron and a neutron. In the alterna- tive possibility where the incident neutrino carries muon the flux of atmospheric neutrinos have found results that flavor, a muon appears in the final state instead. suggest the disappearance of muon-neutrinos when By the 1960s neutrino beams were no longer a futur- propagating over distances of the order of hundreds of istic dream, but had become one of the most important kilometers (or more). Experiments that measure the flux tools of particle physics. Neutrino physics contributed in of solar neutrinos (Bahcall, 1989) suggest the disappear- important ways to the discovery of quarks, the constitu- ance of electron neutrinos while propagating within the ents of protons and neutrons. The technique is concep- Sun or between the Sun and the Earth. The disappear- tually simple, though technologically very challenging. A ance of both atmospheric ’s and solar e’s is most eas- very intense beam of accelerated protons is shot into a ily explained in terms of neutrino oscillations. As con- beam dump which typically consists of a kilometer-long cerns experiments performed with laboratory beams, mound of earth or a 100-meter-long block of stainless most have given no evidence of oscillations. One excep- steel. Protons interact with nuclei in the dump and pro- tion is the Liquid Scintillator Neutrino Detector duce tens of pions in each collision. Charged pions decay (LSND) experiment, which has observed the appear- Rev.
Details
-
File Typepdf
-
Upload Time-
-
Content LanguagesEnglish
-
Upload UserAnonymous/Not logged-in
-
File Pages58 Page
-
File Size-