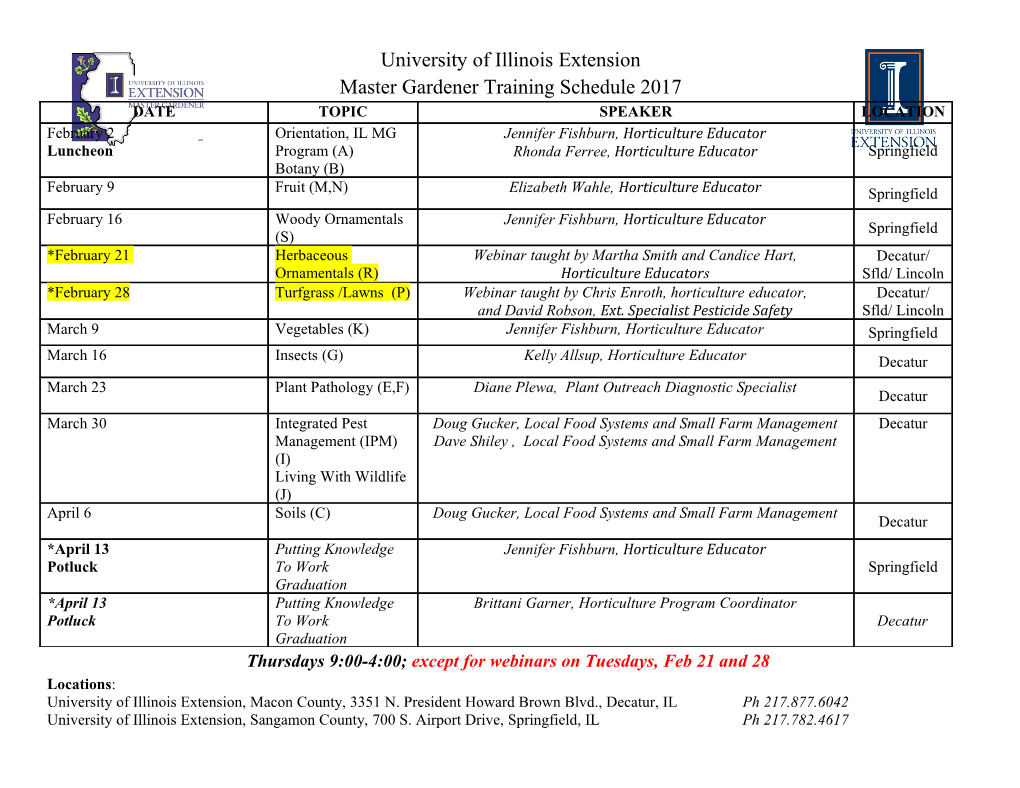
THERMAL METROLOGY TECHNIQUES FOR ULTRAVIOLET LIGHT EMITTING DIODES A Thesis Presented to The Academic Faculty by Shweta Natarajan In Partial Fulfillment of the Requirements for the Degree Master of Science in the School of Mechanical Engineering Georgia Institute of Technology December 2012 Copyright 2012 by Shweta Natarajan THERMAL METROLOGY TECHNIQUES FOR ULTRAVIOLET LIGHT EMITTING DIODES Approved by: Dr. Samuel Graham, Advisor School of Mechanical Engineering Georgia Institute of Technology Dr. Andrei G. Fedorov School of Mechanical Engineering Georgia Institute of Technology Dr. Shyh-Chiang Shen School of Electrical and Computer Engineering Georgia Institute of Technology Date Approved: October 18th, 2012 To my parents – Indira and Ram Natarajan, my grandparents – Gowri and P.K. Murthy, and my husband Ashok Rajendar ACKNOWLEDGEMENTS I am grateful to my advisor, Prof. Samuel Graham, for his guidance, mentorship, support and inspiration over the past few years. Prof. Graham taught me to approach a multi-disciplinary problem from its fundamental roots, and challenged me be a better thinker and a better researcher. It has truly been an honor to work with him. I would like to thank Prof. Andrei Fedorov and Prof. Shyh-Chiang Shen for taking the time to be on my committee. Their guidance is very much appreciated. I would like to thank Prof. Timothy Lieuwen and Dr. Jacqueline O’Connor, for their mentorship and teachings during my time as an undergraduate researcher. I am very fortunate to have worked with Jackie - I have learned much from her about solving scientific problems and about being a successful individual, and for that I am grateful. I would like to thank my supervisor during my internship at 3M, Dr. Karl Geisler. I could not have asked for a better mentor. I have learned a lot from him and am truly grateful for his technical and professional guidance. I would like to thank Sukwon Choi and Dr. Thomas Beechem for their guidance regarding Raman spectroscopy. I would also like to thank all members of the Graham group, past and present, for their support and teachings. I am extremely grateful to Dr. Wayne Whiteman and Ms. Glenda Johnson for their insights about graduate school, and their patience with my problems. Their guidance was invaluable. Over the past few years, I have had the fortune to work with and learn from some truly wonderful people. I would like to thank my good friend Dr. Anusha Venkatachalam for teaching me the basics of my research topic, for her support, and for her professional advice. I am very i grateful to my good friend Yishak Habtemichael for his insights into our research, and his constant cheerful demeanor. I am very grateful to my close friends F. Nazli Donmezer and Dr. Anuradha Bulusu for their technical and personal advice, and for their emotional support. I am grateful to Anne Mallow and Dr. Timothy Hsu for being great friends and officemates. These people really did make graduate school at Georgia Tech a happy experience for me, and I could not have made it this far without their support and companionship. I am very grateful to my best friend, Sweta Vajjhala, for always being there for me. She has taught me the value of true friendship, and I am extremely fortunate to have her in my life. Lastly, I am grateful beyond words to my family. I truly owe all of successes to my parents, grandparents, parents-in-law, sister and husband. Their unconditional love and support motivates me to do better every single day. I cannot thank them enough. ii TABLE OF CONTENTS Page ACKNOWLEDGEMENTS i LIST OF TABLES v LIST OF FIGURES vii SUMMARY xv CHAPTER 1 INTRODUCTION AND MOTIVATION 1 1.1 Introduction to UV LEDs 1 1.2 Operating principles of an LED 8 1.3 Fundamental challenges in the development of DUV LEDs 13 1.4 The Flip Chip package structure for high power visible and UV LEDs 26 1.5 The effect of temperature on UV LED degradation 33 1.6 Research motivation and outline 39 2 UV LED PACKAGING AND LITERATURE REVIEW OF UV LED THERMAL METROLOGY 42 2.1 Packaging schemes for high power LEDs 42 2.2 Metrology techniques for high power UV LEDs and literature review of key studies 47 3 EXPERIMENTAL METHODOLOGY FOR INTERNAL DEVICE TEMPERATURE MEASUREMENTS 74 3.1 Device structure, electrode geometries and measurement locations of investigated UV LEDs 74 3.2 Instrumentation, calibration and measurement procedures for micro- Raman spectroscopy 84 iii 3.3 Instrumentation, calibration and measurement procedure for Infrared spectroscopy 89 3.4 Instrumentation, calibration and measurement procedures for Electroluminescence (EL) spectroscopy 91 3.5 Calibration and measurement procedures using the Forward Voltage method 94 4 EXPERIMENTAL RESULTS AND DATA ANALYSIS FOR INTERNAL DEVICE TEMPERATURE MEASUREMENTS 97 4.1 Experimental results and data analysis for micropixel devices 97 4.2 Experimental results and data analysis for interdigitated devices 127 5 MEASUREMENTS OF THE THERMAL RESISTANCE EXTERNAL TO THE LED 138 5.1 Modified Thermal Resistance Analysis by Induced Transient (TRAIT) method 138 5.2 The results of the modified TRAIT method applied to the ANSYS based FEA of an LED package 149 5.3 The results of the modified TRAIT method applied to the white light LED with an IMS substrate 154 5.4 The results of the modified TRAIT method applied to the white light LED with an SMT substrate 158 5.4 The results of the modified TRAIT method applied to the yellow light LED 162 6 CONCLUSIONS AND FUTURE WORK 166 APPENDIX A 170 APPENDIX B 173 APPENDIX C 176 REFERENCES 177 iv LIST OF TABLES Page Table 3.1 Table describing the four micropixel UV LED devices investigated in this study 77 Table 4.1 Stokes peak shift coefficients from Eq. 2.6 for Locations II and III, in a micropixel without a hotspot, in the DUVL device, as well as the coefficient of determination of fit for Eq. 2.6 101 Table 4.2 Peak shift coefficients from Eq. 2.6, and coefficients of linear fit in the correction for inverse piezoelectric stress in Eq. 3.2, as well as the coefficients of determination for Eqs. 2.6 and 3.2, for Location I for the micropixel without the hotspot, in the DUVL device 101 Table 4.3 Linewidth method coefficients of fit, from Eq. 3.9, for Locations II and III, in the micropixel with a hotspot, as well as the coefficient of determination of fit for Eq. 3.9 109 Table 4.4 Temperature rise at the hotspot, measured by micro-Raman thermography at Locations II and III, and by IR thermography, at an input power of 400 mW 109 Table 4.5 Micro-Raman peak shift coefficients for the AlN and sapphire layers (Locations II and III) in the micropixel Devices 1, 2, 3 and 4, from Eq. 2.6, as well as the R2 value of fit 111 Table 4.6 Comparison of temperature measured by EL spectroscopy and micro- Raman spectroscopy on Location I of the DUVL 121 Table 4.7 Vf method calibration coefficients from Eq. 2.1 for the micropixel devices 124 Table 4.8 Micro-Raman peak shift coefficients from Eq. 2.6 for the AlN and p-GaN layers in the interdigitated device, along with the R2 of fit 129 Table 5.1 Thermal resistances and capacitances derived from the modified TRAIT method, for the ANSYS based thermal finite element model of packaged LED 152 Table 5.2 Thermal resistances and capacitances derived from the modified TRAIT method, for the white light LED with an IMS substrate 157 Table 5.3 Thermal resistances and capacitances derived from the modified TRAIT method, for the white light LED with SMT power substrate 161 v Table 5.4 Thermal resistances and capacitances derived from the modified TRAIT method, for the yellow light LED 164 vi LIST OF FIGURES Page Figure 1.1 Properties of the electromagnetic spectrum across various frequencies and wavelengths 2 Figure 1.2 A schematic showing the ultraviolet region of the electromagnetic spectrum sub-divided into regions with various nomenclatures based on wavelength range 3 Figure 1.3 (a) DUV LED water disinfection unit showing flow chamber and (b) DUV LED array with stirrer rod, insider flow chamber 5 Figure 1.4 (a) Different applications of DUV LEDs in NLOS systems and their attributes and functions, and (b) first generation DUV LED communication link showing transmitter with LED array, and receiver 6 Figure 1.5 The external quantum efficiency (EQE) of UV LEDs emitting between 200 nm and 400 nm, arranged by research group or organization of origin 7 Figure 1.6 (a) p-n junction of under zero-bias, and (b) p-n junction under a forward bias V, showing narrowing of depletion region 9 Figure 1.7 Schematic of active regions showing multiple quantum wells, the electron blocking layers and the confinement regions in a GaN based LED 11 Figure 1.8 Plot of bandgap energy against lattice constants, for InN, GaN and AlN at room temperature 13 Figure 1.9 Hexagonal wurtzite structure of AlN and GaN, showing crystal orientation and lattice parameters 14 Figure 1.10 Schematic of the interface between two semiconductors with a lattice mismatch, showing the presence of dangling bonds 15 Figure 1.11 (a) Cubic crystals with different lattice constants, a1 and a0, with a1<a0 and (b) coherently strained pseudomorphic semiconductor layers 16 Figure 1.12 (a) and (b) Screw, edge and mixed type dislocations seen in a TEM image of AlN grown on sapphire through MOCVD, viewed under different directions 17 vii Figure 1.13 Demarcated current path, showing current crowding effects, between the p and n contacts in a GaN based LED grown on an insulating substrate.
Details
-
File Typepdf
-
Upload Time-
-
Content LanguagesEnglish
-
Upload UserAnonymous/Not logged-in
-
File Pages206 Page
-
File Size-