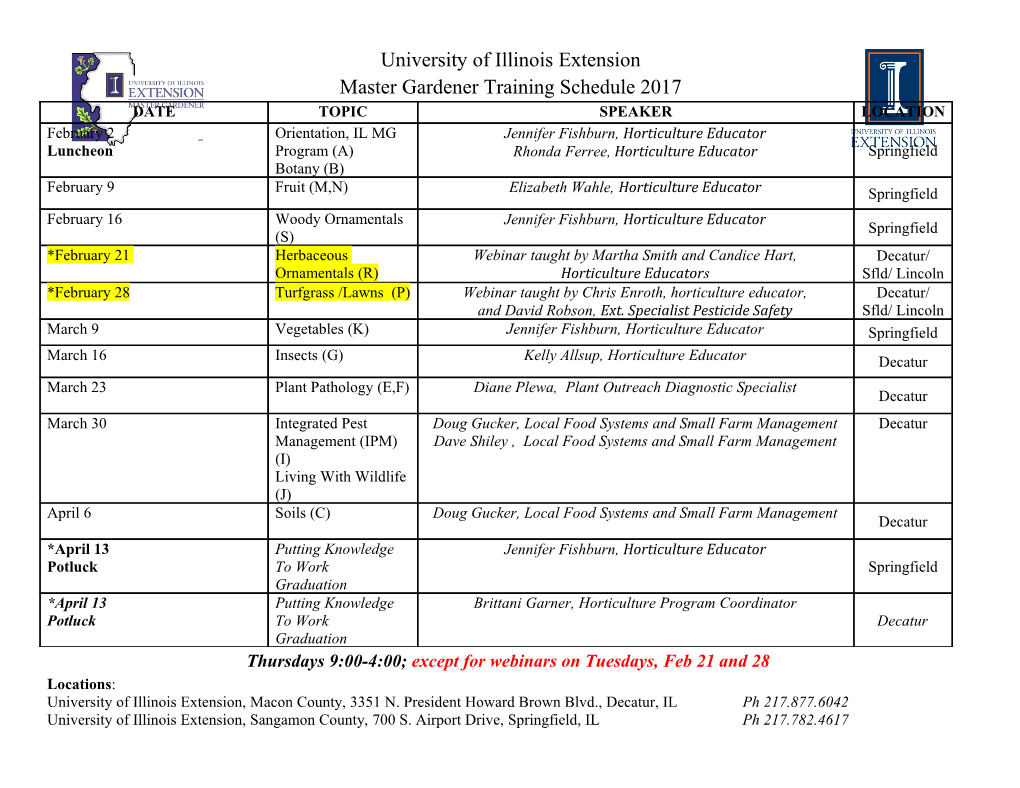
Zeolites fit for a crown: probing host-guest interactions with thermogravimetric methods A. Nearchou, R. Castaing, P. R. Raithby and A. Sartbaeva University of Bath, Department of Chemistry, Claverton Down, Bath, BA2 7AY Abstract Every year millions of tons of zeolites are produced, being used as molecular sieves, hydrocracking catalysts, gas-capture materials and for emerging novel applications. There is a demand to synthesize new zeolites with bespoke frameworks, which are tailor-made for a chosen application. Steps towards tailor making zeolites have been listed in the top ten de-sired breakthroughs in 2011 by Science. To achieve these ‘designer zeolites’ it is crucial to fully understand the host-guest interactions between organic templates, used in their preparation, and the zeolitic frameworks, which are still not known. Here we have studied four different zeolites, synthesized with the same organic template, 18-crown-6 ether, which show observable differences in the host-guest interactions. We demonstrate that the framework dominates the decomposition temperature, enthalpy and mechanism. The zeolites show unique decomposition features, emphasizing, for the first time, the difference in how the template and framework interact during synthesis. Introduction Zeolites are crystalline aluminosilicate minerals, characterised by their unique open framework architectures and periodic microporosity. Currently, zeolites have a variety of uses across the chemical sciences, such as catalysts for organic chemistry, molecular sieving, carbon dioxide capture and many other emerging applications.1-4 To this day, zeolites are still the primary catalyst used for petroleum hydrocracking, demonstrating their contemporary robustness that has not been replaced via alternative porous materials.5 As such, there is a desire to prepare new de-signer zeolites which possess bespoke structures to enhance chosen chemical applications.6-10 Current approaches towards designer zeolites make use of organic additives, which direct the crystallisation of a desired topology. The addition of organics was first reported in 1961 by Barrer et al.11 but it was not until 1967 that the use of additives was utilised to crystallise a new zeolite; high- silica zeolite beta.12 As it is, the use of organic additives is a necessity, being needed to direct the desired structure. An organic additive’s involvement in synthesis consists of non-bonding, van der Waals interactions with the assembling silica oligomers.13-15 These organic-framework host-guest interactions are integral in discriminating which framework is grown.16 Organic additives can be subdivided into space-filling species, organic structure directing agents (OSDA) and true templates, depending on the degree and nature of these interactions.14 One of the methodologies employed to prepare new zeolites is to use computer simulations to predict which additives have the most favourable non-bonding interactions with the framework.15, 17 However, the distinct nature of these interactions in framework assembly are still not known.10 Therefore, to aid our design of new zeolites, it is crucial to understand the role that organic additives play in synthesis. To glean experimental information on the host-guest interactions involved in framework assembly we have studied zeolites Na-X (FAU), EMC-2 (EMT), RHO and ZK-5 (KFI). All four zeolites express unique structures, as shown in Figure 1, however they can all be prepared using 18-crown-6 ether (18C6) as an organic additive. Amongst these four zeolites, incubation times and quantities of Si and Al precursors are similar, with the most striking difference being the cations used in the synthesis.18- 21 Interestingly, all of the zeolites can be prepared without the use of 18C6,22, 23 aside from zeolite EMC-2 where it appears to be a necessity under current techniques.24 Considering cations, zeolites Na-X and EMC-2 are prepared exclusively with Na+, zeolite RHO with Na+ and Cs+, and zeolite ZK-5 with K+ and a small amount of Sr2+. Seeing a difference in cations used, it puts into question collaborative structure directing effects if the cation is coordinated to the cavity of 18C6, and whether this distinguishes which framework is assembled.25 Figure 1│ Polyhedral structures of the topologies of the four zeolite materials studied: zeolite Na-X, zeolite RHO, zeolite ZK- 5, and zeolite EMC-2. Both zeolites RHO and ZK-5 are viewed down the a axis, zeolite Na-X along the (1.2, 1.2, 0) plane and zeolite EMC-2 down the c axis. These structures clearly show the channel geometries with labelled sizes of the pores which are occupied by 18-crown-6 ether.21, 31-33 The chemical structure of 18-crown-6 ether (18C6) is also shown, as well as the approximate molecule size.34 Through a combination of thermogravimetry, mass spectrometry and differential thermal analysis, we demonstrate for the first time that all four zeolites show experimental differences in their host- guest interactions with 18C6. In addition, we have estimated the kinetics of 18C6 decomposition via the methods by Ozawa, Flynn and Wall,26, 27 which is a technique that has not been applied to zeolites previously. Using these findings as a toolkit, a more rational use of OSDAs can be utilised to prepare designer zeolites. Furthermore, the new methodologies presented herein can be applied to current zeolites, such as MFI-type zeolites used in the petrochemical industry. Silicalite-1 and ZSM-5 are MFI-type zeolites that can be synthesised with a variety of organic templates, so it would be beneficial to understand how the different templates interact with the framework.28-30 Experimental Materials The materials used in the synthesis of all four zeolites were colloidal silica (LUDOX® HS-40, 40 wt% SiO2 suspension in water) as a silica source, distilled water as solvent and 18-crown-6 ether (C12H24O6, 18C6) as OSDA. Amongst the syntheses, other materials used include sodium hydroxide (NaOH), potassium hydroxide (KOH), strontium nitrate (Sr(NO3)2) and aqueous caesium hydroxide (CsOH, 50wt% solution in water), as sources of cations and base. The source of alumina was either sodium aluminate (NaAlO2) or aluminium hydroxide (Al(OH)3). All materials were purchased from Sigma-Aldrich aside from the aluminium hydroxide, which was from ACROS. Zeolite EMC-2 Synthesis Synthesis of an EMT-type zeolite material was performed using the method we previously reported,24 which was an adjusted method of preparing zeolite EMC-2 by Chao and Chatelain.22 The molar composition used in the precursor hydrogel was 2.0 Na2O / Al2O3 / 9.7 SiO2 / 0.47 (18C6) / 87 H2O. In a typical synthesis, the sodium hydroxide and 18C6 were dissolved in distilled water. The sodium aluminate was added to this solution and stirred until clear. Slowly the colloidal silica was poured into the solution, allowing gelation. The produced hydrogel was then stirred under ambient conditions for 24 hours before being transferred to a Teflon cup inside a sealed stainless-steel autoclave and being heated for 12 days at 110°C. The crystallised material was then filtered and washed with distilled water until it was of neutral pH. The material was also washed to ensure that no residue 18C6 was left on the crystal external surface. The powder was subsequently dried in a 100°C oven overnight and ground. Zeolite Na-X Synthesis We synthesised a FAU-type zeolite with 18C6 molecules occluded in the pores by using the same method for zeolite EMC-2, but with a larger concentration sodium hydroxide. This was to favour the crystallisation of a zeolite Na-X over zeolite EMC-2. The theory behind this is explained in our previous work concerning EMT-type zeolite synthesis with 18C6.24 Therefore, the molar composition used was 3.0 Na2O / Al2O3 / 9.6 SiO2 / 0.47 (18C6) / 91 H2O. The same procedure for zeolite EMC-2 was used, however hydrothermal treatment was reduced to 8 days. Zeolite RHO Synthesis We prepared zeolite RHO with 18C6 as OSDA following the method reported by Chatelain et al.20 in 1995, which we have also performed previously.35 The molar batch composition of the hydrogel was 1.8 Na2O / 0.3 Cs2O / Al2O3 / 10 SiO2 / 0.5 (18C6) / 100 H2O. The procedure used was the same for both zeolite EMC-2 and Na-X, however the caesium hydroxide solution was added in the same step alongside the sodium hydroxide and 18C6. Also, the hydrogel was under hydrothermal conditions for 8 days. Zeolite ZK-5 Synthesis A high silica KFI-type zeolite analogous to zeolite ZK-5 was synthesised using the method reported by 18 Chatelain et al. in 1996. The molar batch composition of the hydrogel was 2.7 K2O / 0.1 SrO / Al2O3 / 10 SiO2 / 1.0 (18C6) / 220 H2O. The synthesis procedure was started by dissolving the potassium hydroxide in a portion of the distilled water. To this basic solution, the aluminium hydroxide was added and the mixture boiled to allow the aluminium source to dissolve. Once a clear solution was obtained it was cooled to room temperature, with any water lost due to evaporation added to the solution. In a separate vessel, the strontium nitrate and 18C6 were dissolved in the remaining portion of distilled water. The colloidal silica was then slowly added to this solution. Once the silica solution was homogeneous, the alumina solution was quickly added to it. The resulting hydrogel was stirred for 30 minutes, before being transferred to a Teflon cup in a sealed stainless-steel autoclave and heated to 150°C for 5 days. The crystallised solid was filtered and washed with distilled water to make it of neutral pH and to ensure all residual 18C6 was removed. After this, the powder was dried in a 100°C oven overnight and ground. Metal Cation–18C6 Complex Synthesis Isolated complexes of the individual metal cations coordinated to the central cavity of the 18C6 molecule were synthesised.
Details
-
File Typepdf
-
Upload Time-
-
Content LanguagesEnglish
-
Upload UserAnonymous/Not logged-in
-
File Pages17 Page
-
File Size-