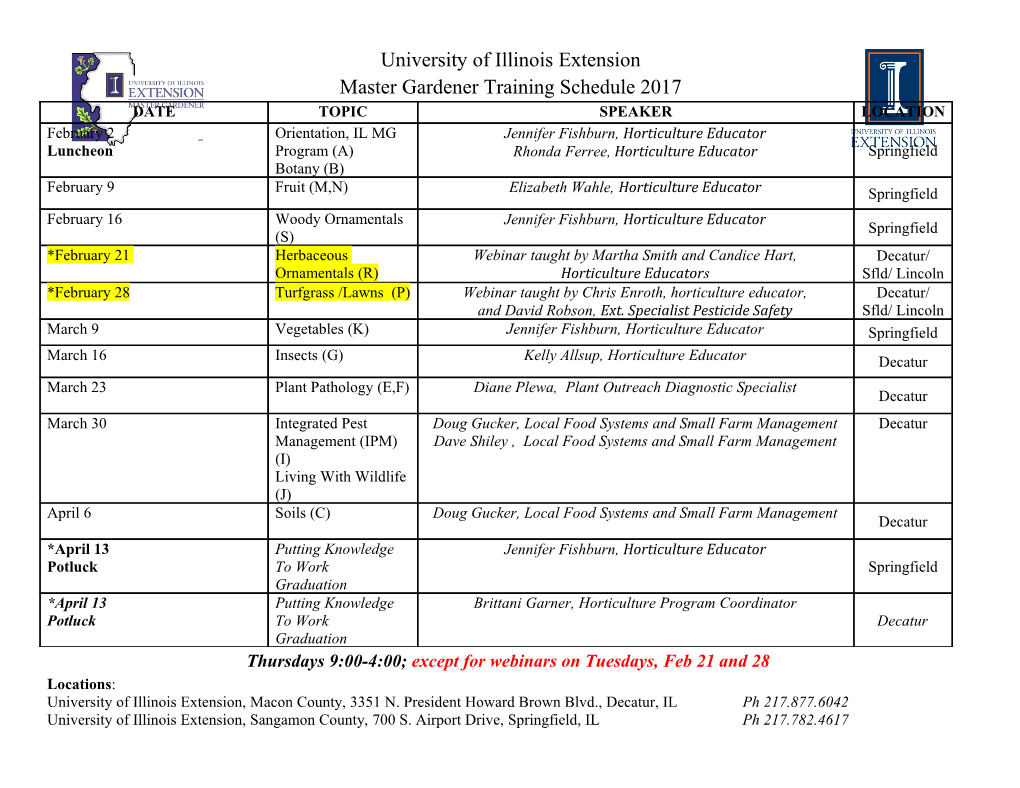
Carnitine metabolism to trimethylamine by an unusual Rieske-type oxygenase from human microbiota Yijun Zhua,1, Eleanor Jamesona,1, Marialuisa Crosattib,1, Hendrik Schäfera, Kumar Rajakumarb, Timothy D. H. Buggc, and Yin Chena,2 aSchool of Life Sciences and cDepartment of Chemistry, University of Warwick, Coventry CV4 7AL, United Kingdom; and bDepartment of Infection, Immunity, and Inflammation, University of Leicester, Leicester LE1 9HN, United Kingdom Edited by David W. Russell, University of Texas Southwestern Medical Center, Dallas, TX, and approved January 29, 2014 (received for review September 5, 2013) Dietary intake of L-carnitine can promote cardiovascular diseases in (14, 15). Assigning functions encoded in the human microbiome humans through microbial production of trimethylamine (TMA) using existing databases can be problematic. For example, the and its subsequent oxidation to trimethylamine N-oxide by hepatic Pfam protein database currently contains over 25% of protein flavin-containing monooxygenases. Although our microbiota are re- families with no assigned functions (release 26.0) (19). sponsible for TMA formation from carnitine, the underpinning mo- Lack of functional characterization of key microbial functions lecular and biochemical mechanisms remain unclear. In this study, in our microbiota is exemplified by very recent studies on car- using bioinformatics approaches, we first identified a two-component diovascular diseases (20–23). These studies have shown that the Rieske-type oxygenase/reductase (CntAB) and associated gene human microbiota is responsible for the production of trime- cluster proposed to be involved in carnitine metabolism in repre- thylamine N-oxide (TMAO), which is believed to promote ath- sentative genomes of the human microbiota. CntA belongs to a erogenesis through its interaction with macrophages and lipid group of previously uncharacterized Rieske-type proteins and has metabolism (20–23). TMAO is derived from the microbial me- an unusual “bridging” glutamate but not the aspartate residue, tabolism of dietary quaternary amines [e.g., choline, L-carnitine, which is believed to facilitate intersubunit electron transfer be- glycine betaine (GBT), and phosphatidylcholine] to trimethyl- tween the Rieske center and the catalytic mononuclear iron center. amine (TMA), which is subsequently oxidized to TMAO by Using Acinetobacter baumannii as the model, we then demon- the host hepatic flavin monooxygenases (21, 22). L-Carnitine MICROBIOLOGY strate that cntAB is essential in carnitine degradation to TMA. Het- (hereafter referred to as carnitine, unless otherwise specified) erologous overexpression of cntAB enables Escherichia coli to is considered an important nutrient for human health, playing produce TMA, confirming that these genes are sufficient in TMA a key role in mitochondrial fatty acid β-oxidation (24, 25). Al- formation. Site-directed mutagenesis experiments have confirmed though carnitine can be acquired through endogenous bio- that this unusual “bridging glutamate” residue in CntA is essential synthesis, our daily demand is largely met through dietary intake in catalysis and neither mutant (E205D, E205A) is able to produce from carnitine-containing food (26). It is known that a significant TMA. Taken together, the data in our study reveal the molecular proportion of dietary carnitine can be further metabolized by and biochemical mechanisms underpinning carnitine metabolism microbiota before absorption (20, 27). This microbial-mediated to TMA in human microbiota and assign the role of this novel metabolic pathway not only diverts carnitine away from the host, group of Rieske-type proteins in microbial carnitine metabolism. causing conditional carnitine deficiency in certain human pop- ulations, but promotes TMAO formation and subsequent in- methylated amine metabolism | comparative genomics | gut microbiota creased risk of atherosclerosis (20, 21). However, the underlying t is increasingly clear that the human microbiota plays an es- Significance Isential role in our health and disease (1–5). Understanding the metabolic potential of the human microbiota and its interaction Metabolism of L-carnitine, a compound abundant in human with and regulation by the host and the environment holds the diet, to trimethylamine by human microbiota has been shown key to unravel the dynamic relationship between ourselves and to promote atherosclerosis and subsequent development of our associated microbes (4–6). Over the last decade, our knowledge heart disease. However, the underpinning molecular and bio- of the human microbiota has been significantly improved thanks to chemical mechanisms remain unknown. In this study, we reveal technological advances, including high-throughput sequencing, de- that a previously unidentified Rieske-type protein is responsible velopment of powerful bioinformatics, and the use of germ-free for carnitine transformation to trimethylamine from human animal models (7–13). We can now not only characterize the tax- microbiota. Knowledge gained in our study provides the op- onomic composition, species richness and dynamics, but com- portunity not only to explore Rieske protein inhibitors in pre- bine the genetic potential encoded in human microbiota and venting trimethylamine formation in animal studies and clinical establish the core metabolic pathways enabled by direct se- trials, but also for its use as a functional genetic marker to quencing of the human microbiome (14, 15). better understand human microbiota and their dynamics in Advances in our understanding of the microbiome functions, our health and disease in future epidemiological studies and however, do not match the pace of taxonomic characterization dietary interventions. of species diversity and dynamics (16–18). Fully resolving the functional capacity encoded in the human microbiome and the Author contributions: Y.C. designed research; Y.Z., E.J., M.C., and Y.C. performed re- search; Y.Z., E.J., M.C., H.S., K.R., T.D.H.B., and Y.C. analyzed data; and Y.Z., E.J., M.C., dynamic effects on health and disease still remains a great H.S., K.R., T.D.H.B., and Y.C. wrote the paper. challenge (11, 16–18). Direct sequencing of the human micro- biome generates datasets dominated by genes encoding pathways The authors declare no conflict of interest. for central metabolism (e.g., transcriptional and translational This article is a PNAS Direct Submission. machinery, ATP synthesis, and so forth) (14, 15), therefore Freely available online through the PNAS open access option. contributing little to our understanding of the variable functional 1Y.Z., E.J., and M.C. contributed equally to this work. capacity encoded in human microbiota between individuals (7, 2To whom correspondence should be addressed. E-mail: [email protected]. 8). Furthermore, a large fraction of the genes encoded in the This article contains supporting information online at www.pnas.org/lookup/suppl/doi:10. human microbiome remain to be functionally characterized 1073/pnas.1316569111/-/DCSupplemental. www.pnas.org/cgi/doi/10.1073/pnas.1316569111 PNAS Early Edition | 1of6 Downloaded by guest on September 29, 2021 genetic and biochemical mechanisms of carnitine-dependent In this report, we describe the identification of the genetic and TMA production in human microbiota have not been uncovered. biochemical mechanisms for TMA production from carnitine in A B Klebsiella pneumoniae subsp. pneumoniae MGH 78578; subsp. pneumoniae HS11286; subsp. rhinoscleromatis ATCC 13384 Klebsiella pneumoniae 342; NTUH-K2044; KCTC 2242 Klebsiella sp. 1_1_55; sp. MS 92-3; sp. 4_1_44FAA CaiT CntA CntB Acinetobacter baumannii ATCC 19606; sp. 6013150; sp. 6013113; sp. 6014059 Acinetobacter sp. SH024; sp. RUH2624 Escherichia coli SE11; MS 198-1; MS 84-1; MS 115-1; Oxygenase (CntA) MS 182-1; MS 146-1; MS 69-1; MS 187-1; MS 21-1; MS 78-1; MS 116-1; MS 175-1; MS 196-1; MS 124-1; MS 119-1; MS 107-1; MS 145-7; Escherichia sp. 4_1_40B Citrobacter youngae ATCC 29220 Citrobacter freundii 4_7_47CFAA Citrobacter sp. 30_2 Gammaproteobacteria Shigella boydii; Shigella dysenteriae; Shigella sonnei; Shigella sp. D9; Reductase (CntB) Providencia stuartii ATCC 25827 Providencia rettgeri DSM 1131 Achromobacter piechaudii ATCC 43553 Betaprobacteria Sporosarcina newyorkensis 2681 Firmicutes CDAcetylcarnitine Carnitine Acylcarnitine Carnitine transporter hydrolase CaiT OH M64747_XylX + (CH3)3N COO- Carnitine [O] Carnitine oxygenase/ reductase, CntAB OH + (CH3)3N - CntA COO O H 0.1 98 OH + O Trimethylamine (CH3)3N Malic semialdehyde O OH Malic semialdehyde dehydrogenase OH O HO Q44256_CbaA Malic acid OH O Malate dehydrogenase Group I To the tricarboxylic acid cycle Fig. 1. Identification of the putative Rieske-type protein from human microbiota in carnitine-dependent TMA formation. (A) Putative carnitine-to-TMA gene cluster in representative genomes of human microbiota. CaiT, carnitine transporter; CntA, A Rieske-type oxygenase protein; CntB, a predicted reductase with + a plant-type ferridoxin domain. (B) Analyses of conserved domains in CntA and CntB. FAD: flavin adenine dinucleotide; NAD : nicotinamide adenine di- nucleotide. (C) An unrooted phylogenetic tree (∼305 aa) of CntA, microbial Rieske-type terminal oxygenases (groups I–IV) and eukaryotic Rieske-type choline monooxygenases. Microbial Rieske-type terminal oxygenases (groups I–IV) are classified based on the nomenclature system of Nam et al. (57). These sequences are identified by a unique GenBank or PDB accession number followed
Details
-
File Typepdf
-
Upload Time-
-
Content LanguagesEnglish
-
Upload UserAnonymous/Not logged-in
-
File Pages6 Page
-
File Size-