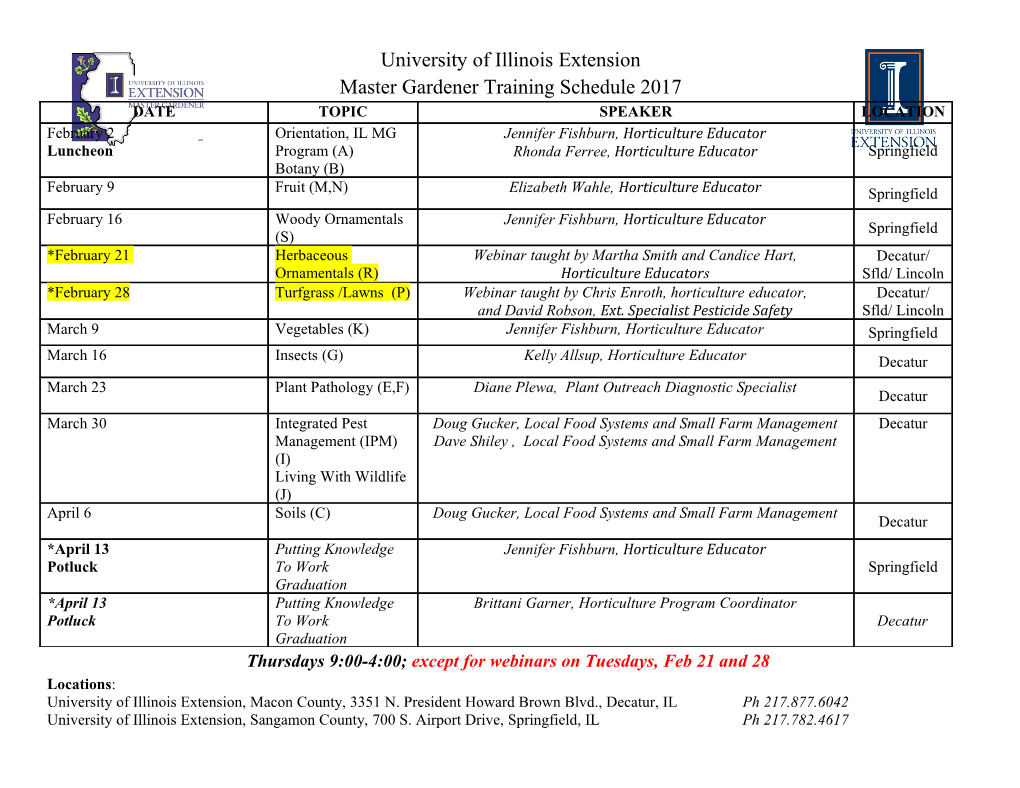
PROPOSAL TO REPRESENT NEUTRON ABSORPTION BY FISSION PRODUCTS BY A SINGLE PSEUDO-FRAGMENT A.M. Tsibulya, A.L. Kochetkov, I.V. Kravchenko and M.N. Nikolaev Abstract The concentration of fission products during reactor operation is analyzed. The dependence of a composite fission product capture cross-section as a function of time and on the nature of the A of the fissile nuclide are investigated, and the neutron radiative capture in fission products of a thermal reactor is evaluated. It is concluded that neutron absorption by fission products can be described by pseudo-fragments. In fast neutron reactor calculations it is customary to represent the absorption of neutrons by fission products by replacing the effect of individual fission fragments by that of a few collective pseudo-fragments. Thus, in the BNAB [1] multigroup data library, the fission product group data for the fissile nuclides mU, mU and 239Pu are obtained by averaging the cross- sections of stable or long-lived (for Ti, larger than 10-100 days) isotopes from each individual decay chain weighted by the fission yield of the corresponding chain. At energies below 160 keV, the contribution from the poisoning isotopes (i.e., isotopes which have a very large thermal neutron absorption cross-section, such as mCd, U9Sm, mSm, 15SGd and 151Gd), whose concentrations depend on the irradiation history of the fuel, are not included in the pseudo-fragment cross-sections. In the calculation of thermal reactors, the cross-sections of the poisoning isotopes as well as those of the radioactive n5Xe, whose concentrations are determined at the time of fission, must be added to the cross- sections of the nuclear ash in the BNAB data library. In 1962, at the time when the BNAB data library was being developed, the composite fast neutron capture cross-sections for the fission products were known to approximately 20-30%. In view of such large uncertainties, it did not seem sensible to consider all individual fission products in fast reactor calculations, and each fission product, with the exception of the poisoning 119 isotopes, in the calculation of thermal reactors. Since then, the accuracy of fission product cross-sections has improved considerably: such that the uncertainty of the composite fission product fast neutron capture cross-sections barely exceed 10%. In view of this situation, the question arises whether it is sensible to describe the interaction of neutrons with fission products by means of a single pseudo-fragment. The drawbacks of such an approach are obvious. As a result of beta decay, the fission products, from the time of their formation, become gradually more neutron deficient, which causes their composite capture cross-section to increase with time. On the other hand, in a working reactor, those fission products that are high absorbers burn out faster, and are converted to weakly absorbing nuclides, with the result that the composite fission product absorption cross-section decreases with the gradual burnup of the fuel. As a result of these processes, the composite fission product cross-section in the reactor core changes with time, and depends on the reactor operation history. If the amplitude of such variations is smaller than the uncertainty of the composite cross-section (which is determined by the existing uncertainties in the knowledge of the neutron data), then the description of the interaction of neutrons with fission products by the characteristics of a single pseudo-fragment is feasible. If this is not the case, then it is then necessary to devise more differentiating means and consider the effects of the individual fission products. Analysis of the evolution of the composite capture cross- section of fission products. The value of the composite fission product capture cross-section as a function of time and degree of fuel burnup was investigated by studying this effect in the core of a 800 MW-electric fast reactor power station. The integral neutron spectrum in the core of the reactor was calculated using the 26-group BNAB-78 [2] cross-section library. The isotopic composition of the irradiated fuel material (including the fission products) was calculated using two independent computer programs.- YIELD [3] and AFPA [4]. These two programs differ in the algorithm used to solve the isotope kinetics equation, in the number of isotopes 120 included in each isobaric chain, in the procedure used to construct the 26-group neutron cross-section sets for the nuclides being depleted and created in the fuel burnup process, and in the manner in which the various fission product yield and decay chain data compilations were used. Both programs were used to calculate the time dependence of the average fission product cross-section during four reactor operating cycles without interruption for fuel loading. Each cycle consisted of an operating time of 110 days at a power level corresponding to a 190 kW/kg thermal load on the unirradiated fuel, and a 10 day reactor shutdown time. At the beginning of the first cycle, the reactor was loaded with unirradiated fuel. The isotopic composition of the fuel at the beginning of the first cycle, and at the end of each irradiation cycle is tabulated in Table I. In addition, the fuel composition at the beginning and at the end of each operating cycle during a continuous reactor operation with a regular fuel reloading of 1/4 of the fuel (that had been irradiated in the course of four cycles) with fresh fuel. TABLE I. TIME HISTORY OF FUEL COMPOSITION DURING REACTOR OPERATION (in at%) Unirrad- End of cycle Continuous cycle Nuclides iated fuel First Second Third Fourth Begin End U-235 0.32 0.27 0.23 0.19 0.16 0.25 0.21 U-238 80.88 78.97 77.11 75.30 73.54 78.06 76.23 Pu-238 0. 10 0.09 0.08 0.08 0.07 0.09 0.08 Pu-239 11.28 11.10 10.91 10.71 10.50 11.00 10.80 Pu-240 4.60 4.73 4.84 4.93 5.00 4.78 4.88 Pu-241 2.05 1.74 1.51 1.34 1.22 1.66 1.45 Pu-242 0.77 0.79 0.80 0.80 0.80 0.79 0.80 Trans-Pu - 0.08 0.14 0.20 0.25 0.10 0.17 Fission - 2.23 4.38 6.45 8.46 3.27 5.38 Products Calculations performed with each of the two programs used various versions of the 26-group fission product cross-section sets: one used the data given in reference [5], another differed from the first by modifying the capture cross-sections of the most important fission products according to the data published in 121 300 700 T I day•I Fig. 1. Behavior of the evolution of (a) the composite fission product capture cross-section 6on, said of (b) the uncertainty in the multi- plication factor 6K, as a function of reactor operating time. 1976 by L.P. Abagyan [4], the third was derived from ENDF/B-V [6], the fourth consisted of data taken from the reference JAERI- M-9715 [7], the fifth was a variation of the third in which the capture cross-sections for 30 fission products were replaced by data based on evaluations described in references [8-10] and in reference [11] for data in the region of resolved resonances. The last version of fission product group data evaluation, which was completed towards the end of 1983 at the Institute of Physics and Power Engineering (FED contains a more complete set of differential neutron data information than any of the earlier versions. The BNAB-78 data were used in the 2)IU, J39Pu and 24tPu burnup calculations, the data by Antsipov et al., [12] for the burnup of 122 mPu and H!Pu, and the BNDF/B-V [6] data for the burnup of the trans-plutonium nuclides. Fission product yield and decay scheme data were taken from the compilation made at the MIFI (institute) [4], the evaluation published in reference [13], and the Japanese compilation [7] based on the yield evaluation published in reference [14]. The calculations were made with yields for both thermal as well as fast neutrons. The isotopic compositions of the fuel and of the fission products, as well as the composite fission product capture cross-sections, were calculated with the various data set versions at a number of time points during each cycle. The results of these calculation, made with different data input, proved to be comparable. These results are included in the cross-hatched region shown in Fig. 1. which can be considered to be the uncertainty envelope of these data. At the very beginning of the first irradiation cycle, when the concentration of the fission products is still very low, the fraction of relatively short-lived neutron rich nuclides is rather large. Therefore the composite fission product capture cross-section at this stage is lower by 7% than it is during the steady-state operation. As one approaches the middle of the irradiation cycle, the neutron rich nuclides which were formed in the beginning of the cycle, have already decayed to stable or long-lived nuclides which are characterized by high capture cross-sections. Neutron-rich nuclides which are continued to be generated by fission, against the background of the earlier fission products, represent by now a smaller fraction. As a result of these processes the composite capture cross-section tends to rise. During reactor shutdown, because of the growth in the population of new fission products and the existing radiation capture capacity of the accumulated fission products, the enrichment of fission products by neutron-rich nuclei ceases; this results in a relatively fast increase in the value of the composite capture cross-section.
Details
-
File Typepdf
-
Upload Time-
-
Content LanguagesEnglish
-
Upload UserAnonymous/Not logged-in
-
File Pages20 Page
-
File Size-