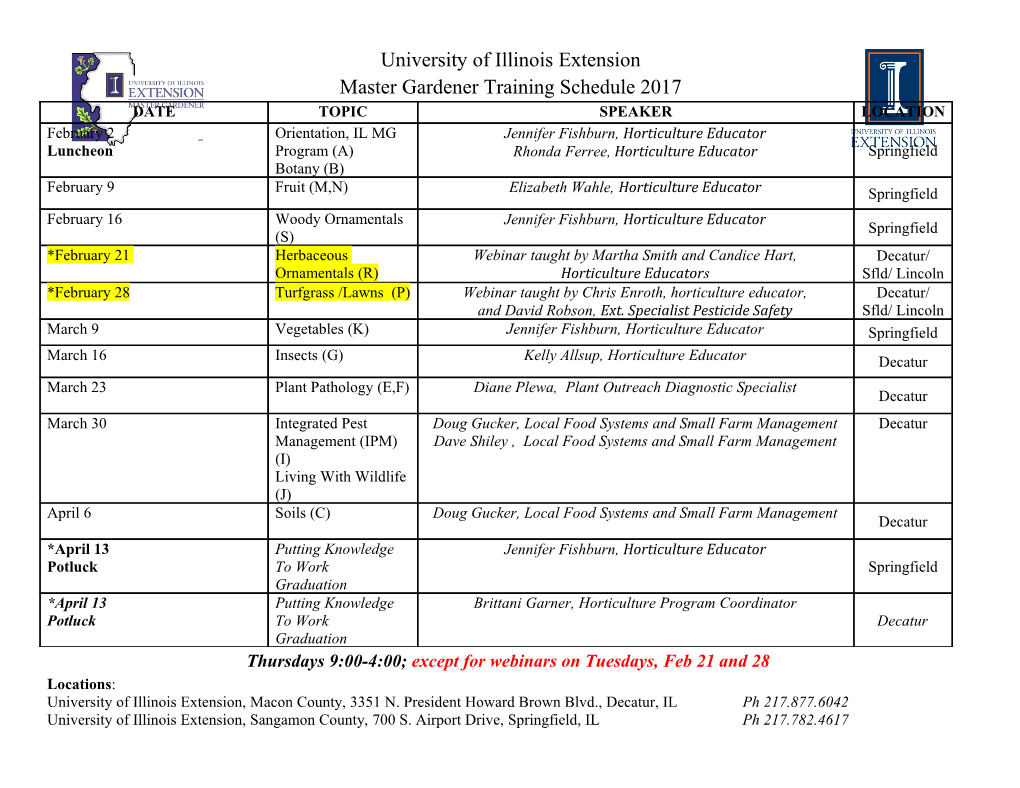
THE ANELASTIC STRUCTURE AND DEEP GEOLOGYOF THE DERBYSHIRE DOME FROM HIGH FREQUENCYRAYLEIGH WAVES by Andrew James William McDonald (BSc, The University of Leeds)' Submitted in accordance with the requirements for the degree of Doctor of Philosophy The University of Leeds Department of Earth Sciences July 1984 i ABSTRACT This work is an investigation of the anelastic structure of the Derbyshire Dome and adjacent areas from the analysis of high frequency, 0.6 - 4.2 Hz, fundamental mode Rayleigh waves observed from quarry blasts within the study area. Seismic field experiments, designed and implemented to record Rayleigh waves accurately, are discussed together with the data preparation sequence. The effects of possible errors on timing and amplitude measurements are investigated and reduced. Time domain measurements indicate the Carboniferous Limestone district of the Derbyshire Dome consists of smaller provinces the boundaries of which are marked by sudden changes in group velocity and co-incide with geological surface features. Similar provinces are not found in the adjacent Millstone Grit district. Approximate estimates of group velocity are higher over the limestone than over the Millstone Grit district. Transformation into the frequency domain facilitates the determination of group arrival times and amplitude spectrum of each Rayleigh wave recorded. Least squares interstation analyses provide estimates of group slowness dispersion for the districts and component provinces and the Rayleigh wave specific attenuation factor, Q-1(v), as a function of frequency for certain districts and provinces. 1, Group slowness which ranges from 0.33 - 1.1 s km increasing generally with frequency, is higher in the Millstone Grit district. Qý1 (v) ranges between 0.01'and 0.08 showing no marked correlation with surface lithology. Models of shear wave velocity, ß(z), and intrinsic specific 11 attenuation factor for shear waves, Qßl(z), with depth, z, are obtained for the upper 1.5 km of the crust in each district and province from the inversion of the observed dispersion and Q-1(v) Y data using linearised and Hedgehog techniques for ß(z) and similar methods for Qßl(z). The deep geology of the area is inferred from the ß(z) models. The limestone district is divided by a major basement fault, to which surface features may be related. The Carboniferous Limestone of different provinces is underlain directly by Ordovician mudstones or Devonian sandstones. A sedimentary basin, of 1.5 km thickness, displaying rhythmic sedimentation is postulated within the Millstone Grit district. Fluid saturation conditions are inferred from the Qß1(2) models. On comparison laboratory Q1 with previous work values of ß which exhibit a possible frequency dependence imply partial saturation of the upper layers of the limestone district whilst 1 field estimates of Qß- not exhibiting any frequency dependence imply the Millstone Grit district to be fully saturated at all levels. Two mechanisms of dissipation based on the petrographic character of the rock and fluid flow are believed to operate jointly. 111 List of Contents. ABSTRACT List of Contents. iii List of Figures. vii List of Tables. xxiv Key to Symbols and Abbreviations. xxv ACKNOWLEDGEMENTS xxvii Chapter One. INTRODUCTION 1 1.1 THE PROJECT. 1 1.2 DEFINITION of ANELASTIC PARAMETERS. 5 1.3 ATTENUATION. 13 1.3.1 Mechanisms of Dissipation. 13 1.3.2 Laboratory and In Situ Determinations of Q-1 16 1.4 SURFACE WAVES and the STUDY of ANELASTICITY. 18 1.4.1 Previous Studies of Surface Waves. 18 1.4.2 The Advantages of Using Surface Waves. 25 1.5 GEOLOGICAL and GEOPHYSICAL SETTING of the REGION. 26 1.5.1 Introduction. 26 1.5.2 Petrology. 27 1.5.21 Carboniferous Limestone. 27 1.5.211 Upper Carboniferous Clastic Sediments. 28 a) Millstone Grit: 28 b) Coal Measures: 29 1.5.2111 Permo-Triassic Sediments. 30 1.5.2iv Igneous Rocks. 30 iv 1.5.3 Sub-Carboniferous Basement. 30 1.5.4 Structural Geology of the Region. 34 1.5.5 Previous Geophysical Investigations. 36 1.5.5i Seismic Surveys. 36 1.5.5 11 Gravity and Magnetic Surveys. 42 1.6 SUMMARY. 43 Chapter Two. EXPERIMENTAL DESIGN, ACQUISITION and PREPARATION of the DATA and PRELIMINARY TIME DOMAIN OBSERVATIONS 45 2.1 INTRODUCTION. 45 2.2 FIELD EXPERIMENTS. 45 2.2.1 Experiment Design. 45 2.2.2 Instrumentation. 49 2.2.3 Field Work. 55 2.2.4 The Source. 57 2.3 DATA PREPARATION. 61 2.3.1 Data Replay. 61 2.3.2 Digitisation. 63 2.3.3 Errors Produced During Data Preparation. 65 2.4 TIME DOMAIN OBSERVATIONS. 71 2.4.1 The Carboniferous Limestone District. 72 2.4.2 The Millstone Grit District. 81 2.5 SUMMARY. 89 Chapter Three. ESTIMATES of DISPERSION and ATTENUATION from HIGH FREQUENCYRAYLEIGH WAVES 92 3.1 INTRODUCTION. 92 3.2 DETERMINATION of ANELASTIC PARAMETERS. 93 3.2.1 The Determination of Group Velocity by the Multiple V Filter Technique and the Calculation of Rayleigh Spectra. 93 3.2.2 Determination of Provincial Rayleigh Wave Dispersion and Attenuation. 101 3.3 ESTIMATES of OBSERVED DISPERSION and ATTENUATION. 105 3.3.1 The Carboniferous Limestone District. 105 3.3.2 The Millstone Grit District. 121 3.4 COMPARISONof OBSERVED RAYLEIGH WAVE DISPERSION and ATTENUATION. 135 3.5 SUMMARY. 141 Chapter Four. INVERSION of OBSERVED RAYLEIGH WAVE DISPERSION 143 4.1 INTRODUCTION. 143 4.2 TECHNIQUES for the INVERSION of OBSERVED DISPERSION DATA. 145 4.2.1 Linearised Inversion Method. 145 4.2.2 Hedgehog Inversion Method. 148 4.3 INVERSION of the OBSERVED RAYLEIGH WAVE DISPERSION DATA. 150 4.3.1 The Carboniferous Limestone District. 155 4.3.2 The Millstone Grit District. 171 4.4 INTERPRETATION of the SHEAR WAVE VELOCITY-DEPTH PROFILES. 180 Chapter Five. INVERSION of OBSERVED RAYLEIGH WAVE ATTENUATION DATA 190 5.1 INTRODUCTION. 190 5.2 TECHNIQUES for the INVERSION of OBSERVED ATTENUATION DATA. 192 vi 5.2.1 Linear Inversion Method. 192 5.2.2 Linear Programming Method. 194 5.3 INVERSION of the OBSERVED RAYLEIGH WAVE ATTENUATION DATA. 196 5.3.1 The Carboniferous Limestone District. 199 5.3.2 The Millstone Grit District. 211 5.4 DISCUSSION of the INTRINSIC SHEAR WAVE ATTENUATION-DEPTH PROFILES. 217 Chapter Six. CONCLUSIONS 229 6.1 THE PROJECT -a resume. 229 6.2 SUMMARY of RESULTS. 235 6.3 SUGGESTED FURTHER WORK and CONCLUDING COMMENTS. 245 Appendix A. INSTRUMENTAL CONSIDERATIONS 251 A. 1 THE EFFECT of the INSTRUMENT on the RECORD. 251 A. 2 INSTRUMENT RESPONSE. 252 A. 3 CALIBRATION. 255 A. 4 INSTRUMENT COMPARISONS. 263 A. 5 CONCLUSIONS on INSTRUMENTATION. 271 Appendix B. OBSERVED RAYLEIGH WAVE DISPERSION and SPECIFIC ATTENUATION FACTOR DATA 273 REFERENCES 281 vii List of Figures. Chapter One. Figure 1.1 Map showing location of the field work area. All maps may be located by the 10 km grid references given which lie in parts of the National Grid squares SD, SE, SJ, SK respectively for this figure and SE and SK for all other maps. ) 2 Figure 1.2 Map of the simplified surface geology, of the region. 31 Figure 1.3 The logs and locations of the deep bore holes of the region. (Carboniferous Limestone outcrop indicated on inset map. ) 33 Figure 1.4 Simplified map of the major structures of the region. (Carboniferous Limestone outcrop indicated. ) 37 Figure 1.5 Disposition and velocity models of previous seismic surveys in the region. Numbers in the velocity models refer to compressional wave velocities in km s'1. (Note the changes of scale for the various models. ) 38 viii Chapter Two. Figure 2.1 Location of stations with identifying experiment mnemonics for the surface wave study. 47 Figure 2.2 Schematic diagram of the field deployment of the basic equipment unit. 51 Figure 2.3 Empirically determined relation between amplifier- modulator (amp-mod) gain setting with distance to produce unclipped signals of optimum amplitude. 53 Figure 2.4 Normalised amplitude response for velocity for the three seismometer types deployed in this study. 53 Figure 2.5 Location of principal quarries in the region in relation to the seismic station lines. (Carboniferous Limestone outcrop indicated. ) 62 Figure 2.6a Schematic diagram of the possible mis-alignment between the recording and replay magnetic heads. 67 Figure 2.6b Time shifts induced by record/replay head mis-alignment shown from the replay of a square wave recorded simultaneously on all channels. 67 Figure 2.7 Comparison of seismograms and amplitude spectra for signals digitised at 25 samples per second (Sps) without anti-aliasing filters (standard format) and ix with anti-aliasing filters having a3 dB point at 12.5 Hz. 70 Figure 2.8 Comparison of seismic sections for two events (F2426, F2525) in Tunstead Quarry recorded along station line LST 1. Signal types 1 and 2 are repeatable and depend on the orientation of the blast to the 74 station. Figure 2.9 Particle motion plot for event F2441 in Brier Low Quarry recorded at station ALL. The seismograms are interpolated to give the same start time; filtered by a Gaussian filter of centre frequency 2.5 Hz and bandwidth ± 2.5 Hz. The horizontal seismograms rotated to give radial (R), transverse (T) components. The vertical (Z) component is retained. The identified phases are discussed in the text. (Positive motion is away from the source for the radial component, upwards for the vertical component and given by a right handed convention for the transverse component. Start of motion in each window marked by *. ) 77 Figure 2.10 Reduced time seismic section for event F2522 in Brier Low Quarry recodred along LST 1. (Amplitudes for all seismic sections are normalised for display purposes. ) 79 X Figure 2.11 Reduced time seismic section for events F2522 in Brier Low Quarry recorded along LST 2.
Details
-
File Typepdf
-
Upload Time-
-
Content LanguagesEnglish
-
Upload UserAnonymous/Not logged-in
-
File Pages349 Page
-
File Size-