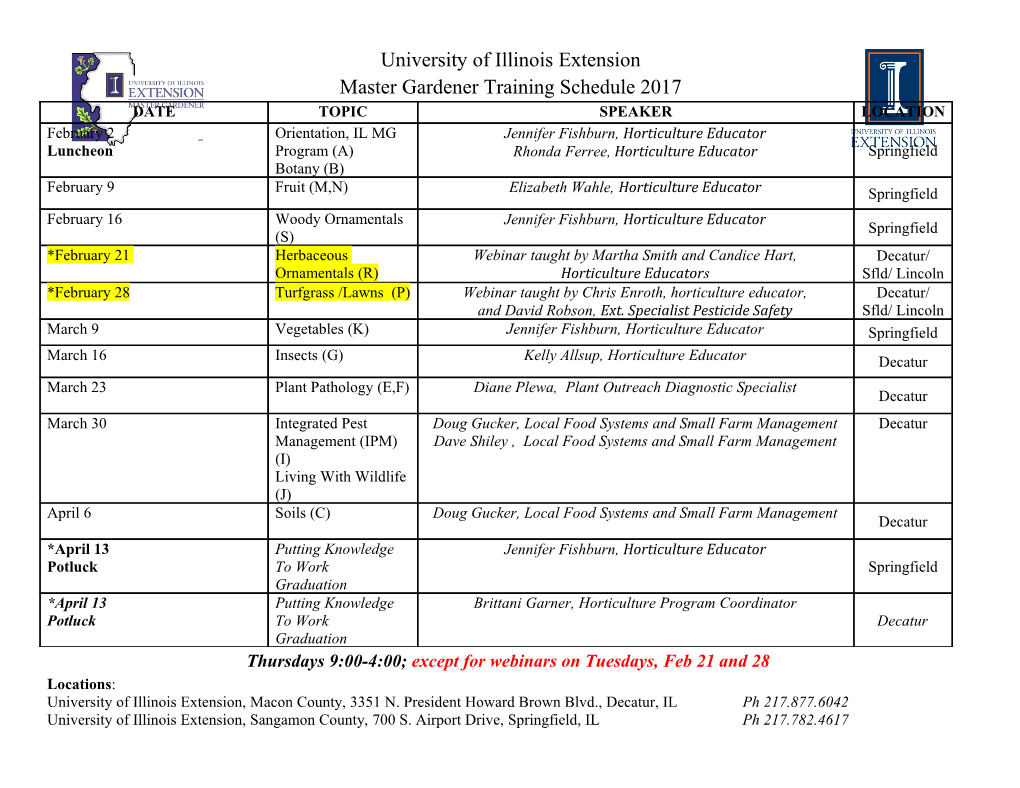
47th International Conference on Environmental Systems ICES-2017-151 16-20 July 2017, Charleston, South Carolina Validation of the Virtual Spacesuit using Apollo 15 Data Claas T. Olthoff1 Technical University of Munich, 85748 Garching, Germany This paper presents results from the validation of a spacesuit simulation system called V-SUIT, which has been under development at the Technical University of Munich since 2012. V-SUIT combines models of spacesuit pressure garments, portable life support systems (PLSS) and a comprehensive human model, with a dynamic model of the thermal environment on an airless planetary surface. This holistic approach enables V-SUIT to depict the complex interactions between spacesuit, human and the environment in several different domains. For the purpose of validating V-SUIT, a model of the Apollo A7LB spacesuit was created and the extravehicular activities (EVAs) performed during the Apollo 15 mission were chosen as a case study. Original flight data recordings were provided by NASA’s Spacesuit Knowledge Capture program. The results of the validation show that V-SUIT is generally capable of reproducing all important aspects of the system’s dynamic behavior. Even though the simulated values do not exactly match the flight data, the trends and time dependent changes are consistent. The differences between flight and simulation data can mostly be explained by the lack of exact technical specifications for the systems that were modeled, the uncertainty associated with metabolic rate as measured during the mission and other modeling inaccuracies. As part of a global sensitivity analysis, the simulations of each of the three EVAs was performed on a model of the actual topography at the landing site, as well as on a flat surface, with identical surface properties and lighting conditions. The resulting data are also presented and discussed. With this successful correlation, V-SUIT now has a reference case that can be used in the analysis of future spacesuit systems, enabling the study of changes to the system configuration like new PLSS components or different pressure garment layups. I. Introduction PACESUITS will be an integral part of future human space exploration missions. These missions and S extravehicular activities (EVA) will be carried out further away from the safe haven of Earth than was the case in past endeavors. There will be limited options for a fast return to Earth, limited access to spare parts and limited communication with experts on the ground due to long communication delays. Additionally, the environmental conditions that may be encountered, be it at the lunar poles, around an asteroid in deep space or on the surface of Mars, will be different and more extreme than the ones in low Earth orbit. When considering the operation of permanent bases on the Moon or Mars, the total number of EVAs that a single spacesuit has to support will significantly increase. As a result, the spacesuits of the future will have to be even more reliable and robust than those of the past. To achieve these goals, the development of new technologies and systems is needed and underway.1 Long duration missions into deep gravity wells, like the Moon and Mars, also drive the development efficient technologies that use fewer 2 consumables than the current spacesuit systems, for example the rapid cycle amine (RCA) CO2 filter or the spacesuit evaporator absorber radiator (SEAR).3 The development of new spacesuits and spacesuit technologies requires among other things a deep understanding of the dynamic behavior of and interactions between the spacesuit, its environment and the human inside. Some of this understanding can be obtained via testing, which is expensive both in financial terms as well as in time. In an effort to provide an additional source of information for spacesuit designers, a dynamic spacesuit simulation tool called the Virtual Spacesuit (V-SUIT) has been developed at the Institute of Astronautics of the Technical University of Munich (TUM). It combines a dynamic simulation model of a spacesuit portable life support system (PLSS) with a sophisticated, dynamic human model and a dynamic thermal simulation of the spacesuit’s environment. This paper 1 Ph.D. candidate, Institute of Astronautics, Boltzmannstr. 15, 85748 Garching, Germany describes the validation of this tool through a comparison of simulation results with spacesuit telemetry data from the extravehicular activities (EVA) performed during the Apollo 15 mission from July 31st to August 2nd 1971. II. Background A. V-SUIT – The Virtual Spacesuit Simply put, V-SUIT is the combination of two tools previously developed at TUM: the Virtual Habitat (V-HAB)4 dynamic life support system (LSS) simulation system and the Thermal Moon Simulator (TherMoS).5 V-HAB has been under development at TUM since 2006 and includes a generic simulation framework for matter, thermal and electrical flows, with a library of standard LSS components. It also includes a comprehensive model of the human physiology6,7 to dynamically provide all of the necessary inputs and outputs to the LSS, both in terms of mass flows and thermal heat flows. V-HAB was successfully validated in 2012 using a model of the International Space Station (ISS) LSS and a set of flight data.8 The main focus of the initial development of V-HAB was to demonstrate the value of, and in some cases the necessity for, dynamic mass flow simulations in contrast to purely static or steady-state LSS analysis methods, especially for systems that include bioregenerative components.9 Similarly, TherMoS was conceived to determine, if dynamic thermal analysis can improve the thermal design of moving spacecraft on the lunar surface, like rovers and astronauts. This movement can cause very rapid thermal changes, for instance by moving from sunlight into the shadow of a boulder and back again in a short period of time. The traditional thermal analysis approach focuses on determining worst cases for hot and cold environments at which the spacecraft will then have to operate at for Figure 1: Geometric long durations. TherMoS uses a ray tracing algorithm and a thermal model of lunar regolith Model of a generic to determine the solar and infrared heat flows at a given location on the lunar surface. spacesuit. These calculations are performed with a time step of one second. As Hager determined, if the solar elevation angle relative to the lunar surface is low, the dynamic thermal environment can have a significantly smaller envelope than the worst hot and cold cases would suggest.10 One of the case studies in Ref. 10 was a spacesuit model. The study, however, only considered the incoming solar and infrared heat flow and not the internal thermal dynamics of the spacesuit itself. This was the starting point for the development of V-SUIT. It combines PLSS technologies modeled in V-HAB with a dynamic thermal simulation of a spacesuit in TherMoS. Reference 11 describes this coupling process in greater detail. In summary, a thermal interface was developed between TherMoS and V-HAB that is based on the exchange of heat flow information for the individual faces of the geometric model of the spacesuit, as shown in Figure 1. Additional models of spacesuit-specific life support system technologies were developed in V-HAB and integrated with the human model into an overall model of a spacesuit. B. Apollo 15 Apollo 15 was the fourth lunar landing performed by the United States. Astronauts Dave Scott (commander) and Jim Irwin (lunar module pilot) touched down at the Hadley Rille landing site on July 30th, 1971. As can be seen from Figure 2, this location provides a complex topography with the Rille itself and some flat terrain surrounded by mountains. Additionally, Apollo 15 was the first mission to feature three surface EVAs (plus one EVA with Dave Scott standing up and looking out of the docking hatch of the lunar module) and the first that utilized the lunar roving vehicle (LRV), or “rover”. These characteristics made it an ideal mission to use as a case study for the validation of V-SUIT. Figure 3 shows a map of the landing site and indicates the three traverses that the two astronauts took during their three excursions. It is important to note, that during EVA 1 and 2, the traverses took the astronauts partially up the base of Hadley Delta, a mountain to the south of the landing site that rises about 3.6 km above the plain.12 In Figure 3 this area is marked as “Apennine Front”. One of the analyses presented in section IVof this paper specifically looks at the thermal influence of this type of dominant topographic feature. 2 International Conference on Environmental Systems Figure 2: Apollo 15 Landing Site at Hadley Rille. Lunar module position is indicated with blue circle. Images taken from references 13 (left) and 14 (right). APOLLO 15 NORTH COMPLEX N EVA 3 10 LM 9A 9 RIMA HADLEY EVA 1 EVA 2 SOUTH CLUSTER 3 EARTHLIGHT BRIDGE 1 DUNE ELBOW 4 2 7 SPUR 6 ST. GEORGE 6A APENNINE FRONT 2 km Figure 3: Apollo 15 Traverse Map. Numbers indicate science stations. Image taken from Ref. 15. 3 International Conference on Environmental Systems III. Simulation Models A. Topography The lunar topography model is created using data from the Lunar Reconnaissance Orbiter’s (LRO) Lunar Orbit Laser Altimeter (LOLA) instrument.16 The processed of extracting and processing this data is discussed in greater detail in ref. 10. The ground resolution of the LOLA data set used to generate the model shown in Figure 4 is 102.7 m. This leads to some imperfections in the model, such as the apparent “end” of Hadley Rille in the bottom left corner of Figure 4. The Rille actually extends further, as can be seen from Figure 4, but here TherMoS interpolates between data points.
Details
-
File Typepdf
-
Upload Time-
-
Content LanguagesEnglish
-
Upload UserAnonymous/Not logged-in
-
File Pages18 Page
-
File Size-