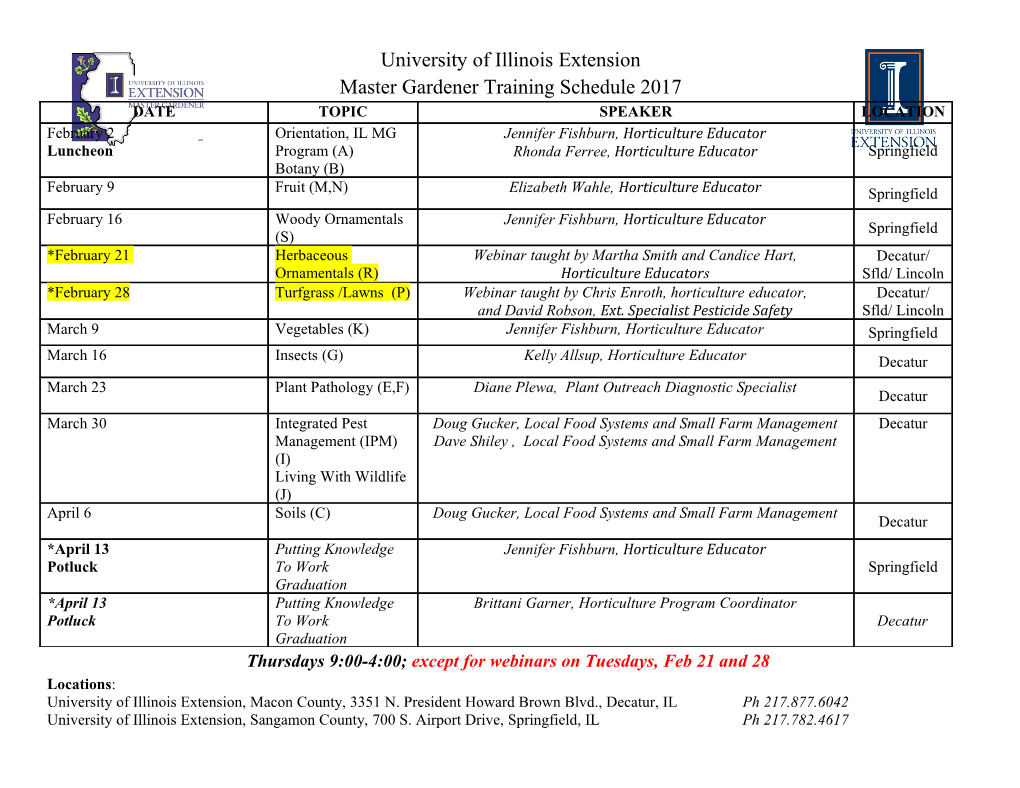
EA41CH16-Eiler ARI 30 April 2013 16:38 The Isotopic Anatomies of Molecules and Minerals John M. Eiler Division of Geological and Planetary Sciences, California Institute of Technology, Pasadena, California 91125; email: [email protected] Annu. Rev. Earth Planet. Sci. 2013. 41:411–41 Keywords The Annual Review of Earth and Planetary Sciences is stable isotope, isotopologue, isotopomer, clumped isotope, online at earth.annualreviews.org position-specific isotope This article’s doi: 10.1146/annurev-earth-042711-105348 Abstract Copyright c 2013 by Annual Reviews. Most natural compounds are composed of diverse isotopologues that dif- All rights reserved fer in the number and/or symmetrically unique atomic locations of isotopic substitutions. Little of this isotopic diversity is observed by conventional Access provided by California Institute of Technology on 01/20/15. For personal use only. Annu. Rev. Earth Planet. Sci. 2013.41:411-441. Downloaded from www.annualreviews.org methods of stable isotope geochemistry, which generally measure concen- trations of rare isotopes without constraining differences in isotopic compo- sition between different atomic sites or nonrandom probabilities of multiple isotopic substitutions in the same molecule. Recent advances in analytical instrumentation and methodology have created a set of geochemical tools— geothermometers, biosynthetic signatures, forensic fingerprints—based on these position-specific isotope effects and multiply substituted isotopologues. This progress suggests we are entering a period in which many new geo- chemical tools of this type will be created. This review describes the princi- ples, background, analytical methods, existing applied tools, and likely future progress of this emerging field. 411 EA41CH16-Eiler ARI 30 April 2013 16:38 1. INTRODUCTION Fractionations of stable isotopes by natural processes are the basis of geochemical tools used to study climate (e.g., Zachos et al. 2001), biogeochemical cycles (e.g., Hedges 1991), and hydrology (e.g., Dansgaard 1964); the origin and evolution of igneous, metamorphic, and sedimentary rocks (e.g., Eiler 2001); the sources of meteorites and other extraterrestrial materials (e.g., Clayton 2007); as well as many other subjects. The precise and accurate methods developed by earth scientists to study subtle natural isotopic variations (McKinney et al. 1950) have led to advances in the use of isotopes in forensics, biomedical science, chemistry, and other disciplines beyond the earth sciences (e.g., Ehleringer et al. 2008). Nevertheless, most of stable isotope geochemistry is based on relatively simple measurements of bulk isotopic composition—an inventory of the proportions of isotopes in a sample, irrespective of their positions within molecular structures or the spatial relationships of rare isotopes with respect to each other. In principle, measurements of the distributions of isotopes in natural materials should provide a diverse, complex, and specific record of their origins, sources, and histories. Consider a familiar organic compound: table sugar (sucrose; C12H22O11)(Figure 1). The substitution of rare for common isotopes (e.g., 13Cfor12C) can take a variety of forms: Approximately 10% of natural sucrose contains a single 13C in one of its 12 carbon positions, and because all the C sites are symmetrically nonequivalent, each of the 12 possible singly 13C-substituted species is unique. Approximately 0.3% of natural sucrose contains at least one deuterium and ∼2% contains at least one 18O. Roughly 0.03% contains both a 13C and a D, and there are 264 geometrically different ways in which this double substitution can be accomplished. When one adds up all possible combinations and spatial configurations of stable isotopes in sucrose, there are ∼3 × 1015 isotopic versions (“isotopologues”) of this molecule—10,000 times the number of stars in our galaxy. Even though many of these combinations are exceedingly rare (some may not even exist in nature), a very large number of singly, doubly, and triply substituted versions have measurable concentrations in the range of parts per million or more—within the reach of modern methods of stable isotopic analysis (e.g., Eiler & Schauble 2004). A full accounting of the isotopic composition of a sample of table sugar, with consideration of only those one-to-severally substituted species that seem potentially analyzable, could involve the most complex isotopic measurement ever attempted. Single substitutions D or 18O or 13C Carbon Oxygen Position-specific Hydrogen 13C differences or Access provided by California Institute of Technology on 01/20/15. For personal use only. Annu. Rev. Earth Planet. Sci. 2013.41:411-441. Downloaded from www.annualreviews.org 18O Nonadjacent D and multiple and D D substitution Adjacent multiple substitution Figure 1 Examples of possible isotopic substitutions in sucrose (C12H22O11). Note not all bonds and atoms are clearly visible in this projection. “Adjacent” multiple substitutions share a bond between the rare isotopes; “nonadjacent” multiple substitutions contain two or more rare isotopes that do not share a bond. 412 Eiler EA41CH16-Eiler ARI 30 April 2013 16:38 Every one of the symmetrically nonequivalent isotopic variants of a molecular structure is unique with respect to its chemical and physical properties (e.g., mass, intramolecular vibration frequencies, moment of inertia, polarizability). Therefore, all such species must generally exhibit variations in relative concentration due to physical, chemical, and biochemical fractionations. Thus, patterns of isotopic substitution—the mix of singly and multiply substituted isotopologues that make up a sample’s comprehensive molecular isotopic composition (here referred to as the sample’s isotopic “anatomy”)—could provide a distinctive forensic fingerprint (Benson et al. 2006), constraints on the sources of substrates from which the molecule was synthesized (Hattori et al. 2011), information regarding the reaction pathways of synthesis (e.g., Monson & Hayes 1982b), the temperature of formation (e.g., Wang et al. 2004), the geographic location of synthesis (e.g., Ehleringer et al. 2008), and perhaps other information. Virtually none of the isotopic diversity we imagine must exist in natural molecular structures has ever been observed through conventional measurements of bulk isotope abundance ratios: A conventional measurement of the isotopic stable composition of sucrose yields only 13C/12C, D/H, 18O/16O ratios, and, possibly, 17O/16O ratios (the latter is presently made in a small number of laboratories). But this situation is changing: An emerging group of novel analytical instruments and meth- ods promises to transform stable isotope geochemistry into a more complete study of isotopic anatomies of molecules and minerals. This emerging discipline has its roots in papers on the chemical physics of isotopes published during the 1930s, and several efforts have examined parts of the problem over subsequent decades. In the past decade, however, the pace of advancement has increased rapidly. This shift has been enabled by technical innovations in isotopic analysis; novel conceptual tools for the geochemical uses of isotopologues; and the emergence of tools with clear value in applied geochemical, geological, and forensic studies. This review examines the history, state of the art, and anticipated future development of the study of position-specific and multiply substituted isotopic compositions of natural materials. Pieces of this subject matter have been addressed in previous reviews (Brenna 2001; Eiler 2007, 2011), but I am not aware of any source that encompasses advances in the various relevant fields— biochemistry, paleoclimate research, atmospheric science, among others. Here, the focus is on a few applied tools that are the leading edge of this discipline: “clumped” isotope analysis of CO2 15 13 and O2; position-specific analysis of NinN2O; and position-specific analysis of CandDin natural sugars, cellulose, vanillin, and other complex organics. However, these are considered to be the earliest proven examples of what is emerging as a frontier of isotope chemistry that will soon reach into other kinds of chemical compounds and impact a wide range of scientific disciplines. 2. MULTIPLY SUBSTITUTED ISOTOPOLOGUES Access provided by California Institute of Technology on 01/20/15. For personal use only. Annu. Rev. Earth Planet. Sci. 2013.41:411-441. Downloaded from www.annualreviews.org In the early 1930s, Harold Urey contributed to several papers on a quantum mechanical theory for isotope effects on the vibrational energies of molecules, starting with H2 (Urey et al. 1932, Urey & Rittenberg 1933, Rittenberg et al. 1934, Urey & Greiff 1935). This work was fundamental to the experimental discovery of D and served as the basis for our understanding of chemical isotopic fractionations generally. This set of short papers (though now rarely cited) is arguably among the most important in all of geochemistry. Several reviews of this theory and its relevance for thermodynamic control of abundances of multiply substituted isotopologues have been presented (Urey 1947, Richet et al. 1977, Wang et al. 2004, Eiler 2007). Briefly, heavy isotope (e.g., D, 13C, 18O) substitution generally reduces vibration frequencies and thus lowers vibrational energies of molecules; this phenomenon is sometimes referred to as the “zero-point energy” effect and is the dominant factor controlling equilibrium stable isotope fractionations. In addition,
Details
-
File Typepdf
-
Upload Time-
-
Content LanguagesEnglish
-
Upload UserAnonymous/Not logged-in
-
File Pages34 Page
-
File Size-