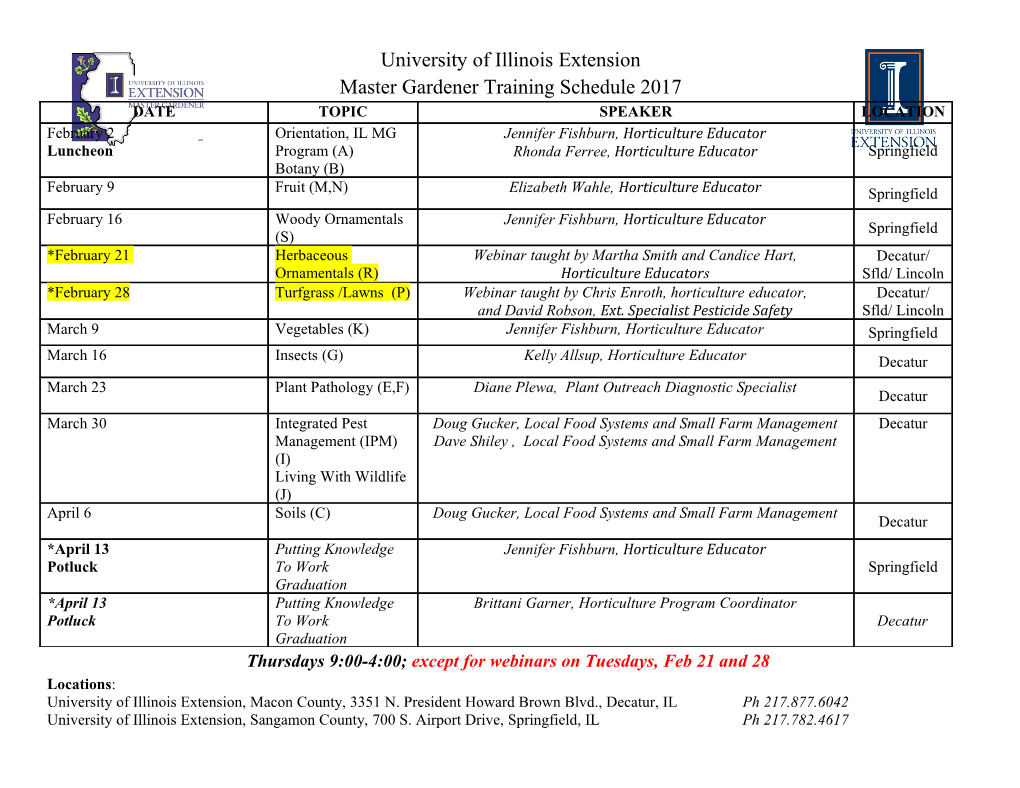
Appendix B Other Approaches to Fusion The main body of this report has discussed magnetic The issues addressed by inertial confinement fusion confinement fusion, the approach to controlled fusion research in the United States concern the individual that the worldwide programs emphasize most heav- targets containing the fusion fuel; the input energy ily. However, two other approaches to fusion are also sources, called drivers, that heat and compress these being investigated. All three approaches are based on targets; and the mechanism by which energy from the the same fundamental physical process, in which the driver is delivered–or coupled–into the target. Due nuclei of light isotopes, typically deuterium and tri- to the close relationship between inertial confinement tium, release energy by fusing together to form heav- fusion target design and thermonuclear weapon de- ier isotopes. Some of the technical issues are similar sign, inertial confinement fusion research is funded among all the fusion approaches, such as mechanisms by the nuclear weapons activities portion of the De- for recovering energy and breeding tritium fuel. How- partment of Energy’s (DOE’s) budget. Inertial confine- ever, compared to magnetic confinement, the two ap- ment research is conducted largely at nuclear weap- proaches discussed below create the conditions nec- ons laboratories; its near-term goals are dedicated essary for fusion to occur in very different ways, and largely to military, rather than energy applications, and some substantially different science and technology a substantial portion of this research is classified. issues emerge in each case. There are two near-term military applications of in- ertial confinement fusion—one actual and one not yet Inertial Confinement Fusion1 realized. First, because the physical processes in a pel- let micro-detonation resemble those in a nuclear The inertial confinement approach to fusion research weapon, inertial confinement experiments now con- has been studied for some two decades, and its cur- tribute to validating computer models of these proc- rent budget almost half that of the magnetic confine- esses, to collecting fundamental data on the behavior ment program. In inertial confinement fusion, a pel- of materials in nuclear weapons, and to developing let of fusion fuel is compressed to a density many times diagnostic instruments for actual nuclear weapons that of lead, and then heated and converted to plasma, tests. These activities can be conducted today with ex- by bombarding it with laser or particle beams (see fig- isting high-energy inertial confinement drivers. ure B-1 ). At this density, about 10 billion times the den- These applications could be greatly intensified, and sity of a magneticalIy confined plasma, the confine- a second set of applications would arise, if a labora- ment time needed is so small (less than one-billionth tory facility producing substantial pulses of inertially of a second) that it shouId be possible to generate net confined fusion energy could be developed. No such fusion power before the pellet blows itself apart. The facility yet exists. Such a facility could simulate the pellet’s own inertia is sufficient to hold it together long effects, particularly the radiation effects, of nuclear enough to generate fusion power, detonations on systems and components. This appli- Inertial confinement already has been demonstrated cation might be particularly important if a Compre- on a very large scale in the hydrogen bomb, an iner- hensive Test Ban Treaty prohibited underground tests tially confined fusion reaction whose input energy is of nuclear weapons. provided by a fission (atomic) weapon. The challenge The near-term, military inertial confinement research of laboratory-scale inertial confinement research is to effort also contributes information that would be es- reproduce this process on a much smaller scale, with sential to any longer term commercial applications. a source of input energy other than a nuclear weapon. For example, both military and civilian applications In a hypothetical inertial confinement reactor, micro- of inertial confinement fusion (other than the com- explosions with explosive yields equivalent to about puter program and diagnostic development activities one-tenth of a ton of TNT would be generated by ir- that are being conducted today) require that an iner- radiating fusion pellets—called targets—with laser or tial confinement target generate several times more particle beams; these explosions would be repeated energy than is input to it. Such an accomplishment, several times a second. which would show the scientific feasibility of inertial fusion, is beyond the capability of any existing lab- ‘The U S inertial con flnem[~nf tuilon rewarch program I\ rm Ie\\ ed I n ,] oratory device. (Of course, thermonuclear weapons recent report by the Nat Ion,l I Rew,] rc h LOU nc I I Ret ~e~t of rhe Dep.] rfment have already demonstrated the scientific feasibility of of f m’rqi s /neflI,I/ Conflnemenf Fu\lon Progr,]rn {Wf,]sh I ngton, DC Nattona I A( .I(l(>rm}, l)rf>~i I W() I very-large-scale inertial confinement fusion. ) 205 206 . Starpower: The U.S. and the International Quest for Fusion Energy Figure B-1.— Inertial Confinement Fusion Process Heating Compression Ignition Burn Laser or particle beams Fuel IS compressed by The fuel core reaches Thermonuclear rapidly heat the surface rocket-like blowoff of high density and ignites. burn spreads of the fusion target, the surface material rapidly through the forming a plasma compressed fuel. yielding envelope. many times the input energy. SOURCE Lawrence Livermore National Laboratory, “ICF Reaction, ” Energy and Technology Review, April-May 1986 The technical requirements for commercial appli- to the reaction chamber, the driver would not have cations of inertial confinement fusion go considerably to be designed to withstand this environment. In the beyond the requirements for weapons effect simula- core of a magnetic confinement fusion reactor, on the tion and would require still further scientific and tech- other hand, systems both for supporting and maintain- nological development. Due to the relatively low ef- ing the plasma and for recovering the energy and ficiencies (e.g., 10 to 25 percent) at which the drivers breeding tritium fuel are located in high radiation, high operate, each target explosion must generate several neutron-flux environments. A second potential advan- times more energy than it is driven with to reach tage of inertial confinement arises from the relatively breakeven. An additional factor of 4 to 10 is required relaxed vacuum requirements inside the reaction beyond breakeven to produce substantial net output. chamber, which would permit the use of neutron ab- In a commercial reactor, therefore, as much as 100 sorbing materials such as liquid lithium inside the first times as much energy must be released in a pellet ex- structural wall of the reactor. Use of such neutron ab- plosion as is required to heat and compress the pellet sorbers would lessen neutron irradiation levels in the to the point where it can react. Furthermore, commer- reactor’s structural elements, increase the lifetimes of cial energy production requires that pellets be deto- those elements, and lessen induced radioactivity nated several times a second, far more frequently than levels. needed for military applications. Finally, cost-effective- On the other hand, inertial confinement also has ness, reliabiIity, and high efficiency are much more disadvantages compared to magnetic confinement. in- important for energy applications than miIitary ones; ertial confinement is inherently pulsed; the systems successful commercialization will depend on how needed to recover energy and breed fuel in the re- well the technology addresses the commercial require- action chamber have to withstand explosions equiva- ments discussed in chapter 5. lent to a few hundred pounds of TNT several times A significant potential advantage of inertial confine- a second. Inertial confinement reactors must focus ment over magnetic confinement is that the complex high-power driver beams precisely on target in this and expensive driver system can be located some dis- environment. Furthermore, the energy gains needed tance away from the reaction chamber. Because ra- for these facilities must be much larger than those of diation, neutron-induced activation, and thermal stress a magnetic confinement device to make up for driver due to the microexplosions could be largely confined inefficiencies. App. B—Other Approaches to Fusion . 207 Four principal driver candidates are now being stud- Cold Fusion ied in the U.S. inertial confinement research program. Two of them —solid-state or glass lasers and light-ion2 Another approach to fusion, presently at an em- accelerators—have by far the largest facilities; the bryonic stage of development, is fundamentally differ- other two principal candidates—gas lasers and heavy ent from either the magnetic or inertial confinement ion accelerators—are in lesser stages of development. concepts. This approach, called ‘‘cold fusion” or Major U.S. glass lasers are located at Lawrence Liver- “muon-catalyzed fusion, ” might make it possible to more National Laboratory in California and the Uni- bypass the requirement for extremely high tempera- versity of Rochester Laboratory for Laser Energetic in tures that make the magnetic and inertial approaches New York. The Livermore facility, the most powerful so difficult.4
Details
-
File Typepdf
-
Upload Time-
-
Content LanguagesEnglish
-
Upload UserAnonymous/Not logged-in
-
File Pages4 Page
-
File Size-