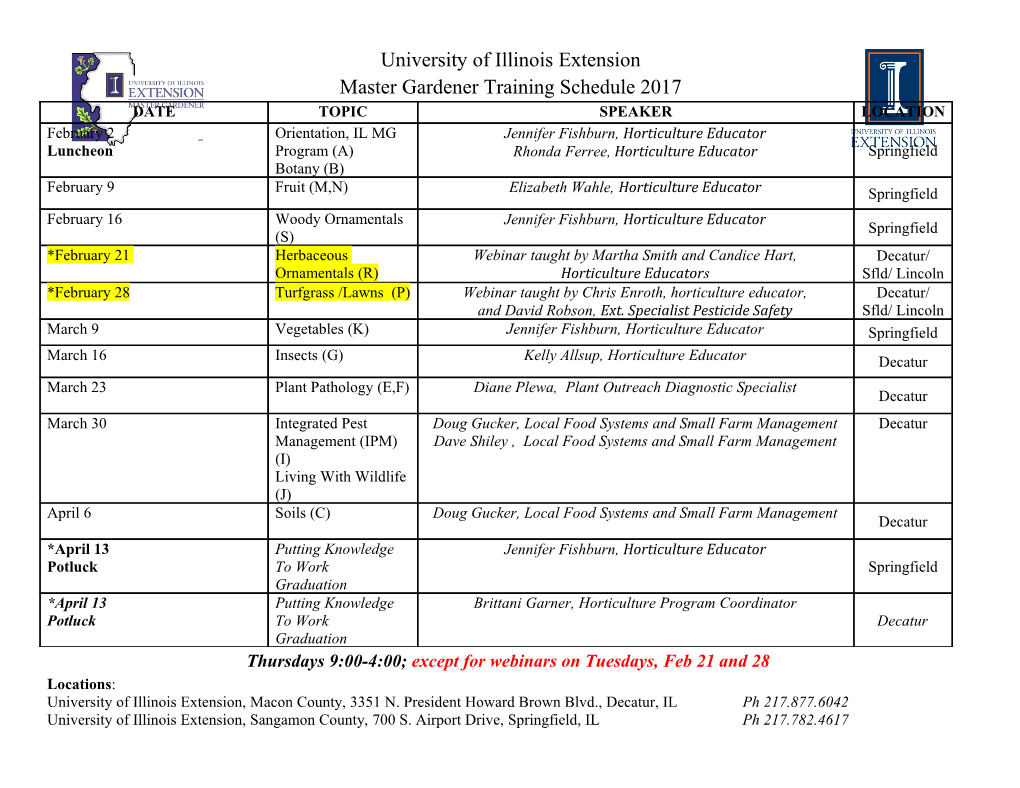
DEGREE PROJECT IN ELECTRICAL ENGINEERING, SECOND CYCLE, 30 CREDITS STOCKHOLM, SWEDEN 2016 Evaluation of two distribution grids in terms of PV penetration limits and effectiveness of reactive power controls LEONARD HÜLSMANN KTH ROYAL INSTITUTE OF TECHNOLOGY SCHOOL OF ELECTRICAL ENGINEERING Evaluation of two distribution grids in terms of PV penetration limits and effectiveness of reactive power controls Leonard Hülsmann Master thesis No. TRITA-EE 2016:170, 2016 KTH Royal Institute of Technology EIT-KIC Energy for Smart Cities Department of Electrical Engineering SE-100 44 Stockholm, Sweden Abstract The large integration of photovoltaic (PV) systems as well as wind turbines in the distribution grid poses new challenges on various levels. One of them is the increased steady-state voltage in the low voltage (LV) as well as the medium voltage (MV) grid, caused by the distributed generation. This can lead to voltage violations above the maximum allowed value of 110% of nominal voltage. There exist various approaches to mitigate this problem. These range from expensive grid reinforcements, like strengthened lines or the installation of on-load tap changers (OLTCs) in distribution transformers, to voltage controls by the distributed generators themselves. One of these voltage controls is the consumption of reactive power by PV inverters. This control can lower the voltage locally but also has an impact on other nodes of the network. A range of possibilities exist, most commonly using an active power dependent reactive power characteristic (cos φ (P) control) or using a voltage dependent reactive power characteristic (Q(U) control). These can either depend on local inputs or involve some kind of coordinated control, for which usually a communication infrastructure needs to be built up. In this thesis, two MV feeders located close to Worms, Germany, have been modelled and validated with measurement data provided by the local distribution system operator. Along the two feeders, a total of three low voltage grids has been modelled in detail. The effectiveness of a local Q(U) voltage control with a deadband has been compared with the worst case, where no reactive power control is applied, and with the best case, where all PV plants maximally participate in the reactive power control. These settings were then tested for different scenarios of increased PV shares. Furthermore, the impact of the Q(U) voltage control on other LV grids, located at the same feeder, has been analysed. The results, in conclusion, demonstrate: i) In general, the examined LV grids have already large PV penetrations and large resulting backflows during times of high PV output. However, the share can still be substantially increased, in particular on LV lines that currently have only low or moderate PV penetration; ii) The Q(U) with deadband regulation can increase the hosting capacity of PV plants typically between 20% and 33% for the investigated networks. The performance can however vary highly between different network configurations and PV distributions; iii) due to the Q(U) control, the reactive power support varies widely between different PV plants in the investigated grids. This decreases the fairness of this method and compensation measures might be needed. However, no quantification of this has been made; iv) The best case shows that good reactive power controls would have a very high potential, so e.g. coordinated voltage controls could significantly increase hosting capacities. 2 Sammanfattning En stor integration av solceller (PV) och vindkraftverk i distributionsnät innebär nya utmaningar på olika nivåer. En av dem är en ökande spänning i lågspänningsnät (LV) samt på mellanspänning (MV), orsakad av distribuerad generering. Detta kan leda till spänningar över högsta tillåtna värde av 110% av nominell spänning. Det finns olika sätt att mildra detta problem. Dessa sträcker sig från dyra nätförstärkningar, som förstärkta linjer eller installation av lindningskopplare (OLTCs) i distributionstransformatorer, till spänningsstyrning av de distribuerade generatorerna. En av dessa spänningsstyrningar är förbrukningen av reaktiv effekt i solcellernas växelriktare. Denna styrning kan sänka spänningen lokalt men den har också en inverkan på andra noder i elnätet. En rad möjligheter finns, oftast med hjälp av en aktiv- effekt-beroende reaktiv effekt (cos φ(P)-styrning) eller med hjälp av en spännings- beroende reaktiv effekt (Q(U)-styrning). Dessa kan antingen styras med hjälp av lokala insignaler eller innebära någon form av samordnad styrning, som vanligtvis medför att en kommunikationsinfrastruktur måste byggas upp. I denna avhandling har två MV-nät, vilka ligger nära Worms, Tyskland, modellerats och validerats med mätdata som tillhandahållits av det lokala distributionssystemets operatör. Längs de två MV-näten, har totalt tre lågspänningsnät modellerats i detalj. Effektiviteten av en lokal Q(U)-styrning med en död-zon har jämförts med det värsta fallet, där ingen reaktiv effektstyrning tillämpas och med bästa fall, där alla PV-omriktare maximalt har deltagit i den reaktiva effektstyrningen. Dessa inställningar testades sedan för olika scenarier för ökad mängd PV. Dessutom har effekterna av Q(U)-styrning på andra LV-nät, som ligger på samma MV-nät, analyserats. Resultaten visar: i) Generellt har de undersökta LV-näten redan stora PV- installationer och stor resulterande back-matning under tider med hög PV-produktion. Mängden PV kan dock fortfarande ökas väsentligt, framför allt på LV-linjer som för närvarande endast har låg eller måttlig mängd PV.; ii) Q(U)-styrning med dödband kan öka möjlig mängden PV med ca 20 - 33% för de undersökta elnäten. Möjlig ökning kan dock variera mycket mellan olika elnät och olika pridning av PV; iii) på grund av den studerade Q(U)-styrningen så varierar den reaktiva effektproduktionen kraftigt mellan olika PV-anläggningar i de undersökta elnäten. Detta minskar en rättvis fördelning med denna metod och kompensations-mekanismer kan behövas. Emellertid har ingen kvantifiering av detta gjorts, iv) Det bästa fallet visar att bra reaktiv effekt-styrning skulle ha en mycket hög potential, så samordnad spännings-styrning skulle avsevärt kunna öka möjlig mängd PV. 3 TABLE OF CONTENTS 1. Introduction ............................................................................................................... 8 1.1. Problem definition ............................................................................................... 8 1.2. Objectives ........................................................................................................... 9 1.3. Overview of the report ........................................................................................ 9 2. presentation of the field .......................................................................................... 10 2.1. Description of passive distribution networks ..................................................... 10 2.2. Impact of distributed generation on the voltage profile in the distribution grid .. 11 2.3. Regulations on steady-state voltage control ..................................................... 13 2.3.1. Voltage limitations .................................................................................... 13 2.3.2. Regulations for generators connected to the MV and LV grid .................. 14 2.4. Voltage control options in distribution networks ................................................ 15 2.4.1. Novel assets and grid planning principles ................................................ 15 2.4.2. Active power control ................................................................................. 17 2.4.3. Reactive power control by distributed generators .................................... 18 2.4.4. The role of communication infrastructure ................................................. 22 2.5. Techno-economical comparison of these voltage control options .................... 22 3. Model description ................................................................................................... 24 3.1. Location and setting ......................................................................................... 24 3.2. Gundersheim substation model ........................................................................ 26 3.3. Overview about available and simulated data .................................................. 27 3.4. Case study #1 – Feeder ‘Gundersheim’ ........................................................... 28 3.4.1. General information .................................................................................. 29 3.4.2. Grid parameters ....................................................................................... 30 3.4.3. Distributed generation .............................................................................. 32 3.4.4. Available measurement data .................................................................... 32 3.4.5. Load modelling ......................................................................................... 34 3.4.6. PV modelling ............................................................................................ 37 3.4.7. PowerFactory implementation .................................................................. 39 3.5. Case study #2 – Feeder ‘Flörsheim-Dalsheim’ ................................................. 41 3.5.1. General information .................................................................................. 41 3.5.2. Grid parameters
Details
-
File Typepdf
-
Upload Time-
-
Content LanguagesEnglish
-
Upload UserAnonymous/Not logged-in
-
File Pages97 Page
-
File Size-