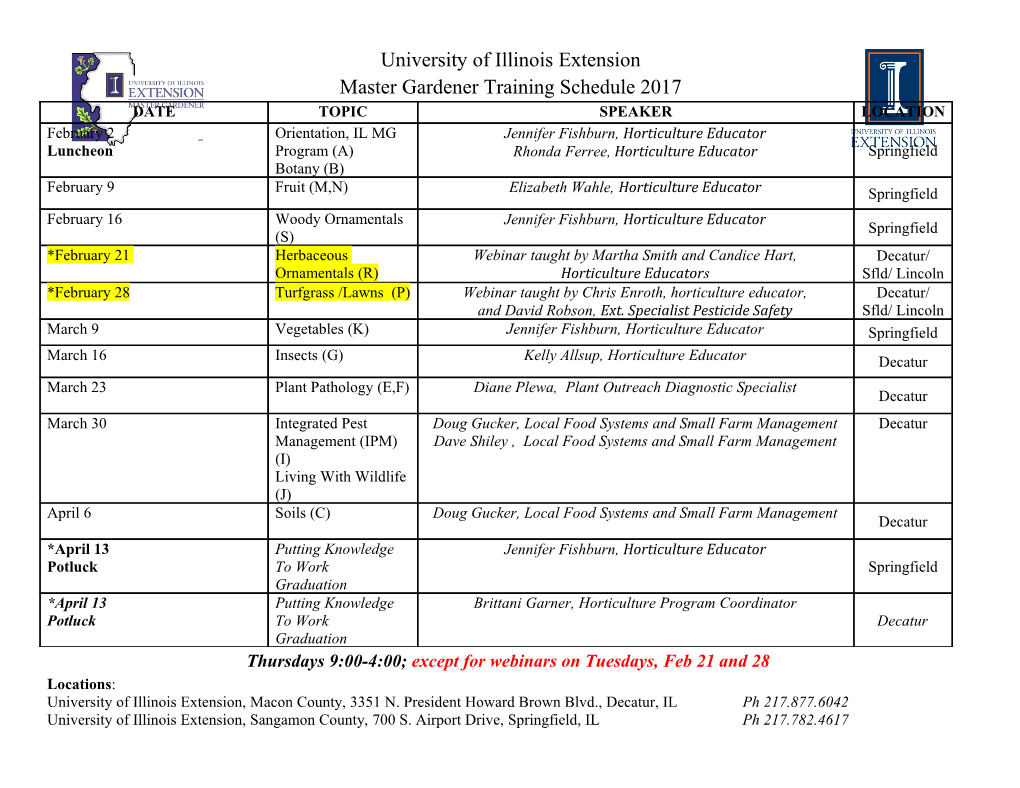
Deepening the Solar/Stellar Connection for a Better Understanding of Solar and Stellar Variability A White Paper Submitted to the Heliophysics Decadal Survey Rachel A. Osten, Space Telescope Science Institute The Sun has had enormous scientific attention paid to it due to its proximity to Earth and the important influences it has on space weather for our increasingly technical society. Yet the Sun is only the closest of billions of stars in our galaxy. And while it has been the subject of daily ground- and space-based observations for decades now, the Sun provides a sample size of one for understanding stellar astrophysics. The primary focus of this white paper is on solar and stellar variability. The purpose is to motivate a deepening of the solar/stellar connection, by recognizing it as a mutual relationship from which both solar physicists and stellar astronomers can benefit. With the impressively detailed nature of solar observations, one may think of the solar/stellar connection as more akin to a one-way street than a multi-lane throughway. However, by exploring the range of behaviors that stars exhibit we can access regions of parameter space which it is not possible to probe with solar observations alone. This white paper addresses four key questions which can be probed with a deeper connection between solar and stellar variability. Question 1: How typical is the Sun as a solar-like star? Solar flares produce emissions across the electromagnetic spectrum, from km- wavelength radio emission to gamma-rays. The interrelationship between various emissions can be used to infer the dynamics and energetics of the flare process, and thus gain insight into the physics involved in flares. Solar white-light or visible flares are difficult to see on the Sun, due to the generally small amount of contrast between the disk-integrated light and the small physical area involved in producing white-light flare emissions. The importance of the white light flare arises from their dominance in the flare energy budget of the radiative emissions from the lower chromosphere and photosphere via UV and white-light continuum emissions (Woods et al. 2006), even if the flare contrast is not as high as in other wavelength ranges (5-30% continuum enhancement in the models of Allred et al. (2005). The frequency of solar flares is distributed in energy according to a power-law in the differential flare frequency distribution dN/dE ∝E-α with α=1.7±0.1 (Kucera et al. 1997), where dN/dE is the flare frequency per unit flare energy. The largest solar flares release about 1032 erg in a matter of minutes (Woods et al. 2004) while a more typical energy release is 1029 erg. The flare frequency is such that the largest events are observed typically only a few times per solar cycle of 11 years. Detailed studies of the energy budget in solar flares and coronal mass ejections (Emslie et al. 2005) led to an estimate that the total solar irradiance as diagnosed in the UV-optical bands indicated total radiated energies roughly 100 times that determined from X-ray studies alone. Studies of stellar variability hold the key to understanding the potential extremes of solar behavior. Infrequent events can be detected much more easily on a large sample of stars than by intense scrutiny of one Sun. For example, determination of flares as large as the Carrington flare on the Sun (Cliver & Svalgaard 2004) are currently suggested to occur every 500 years, but that is an uncertain number. Schaefer et al. (2000) found evidence for superflares on solar-like stars with radiated energies up to 106 times larger than the largest solar flares, with estimated frequencies of once every several hundred years. As human society becomes more technologically advanced, we expose ourselves to increasing vulnerability to solar behavior. Having a better sense of the frequency of such events lets us know what precautions might be needed. The Kepler mission currently operating has a primary mission of finding eclipsing extrasolar planets, but with a monitoring campaign of >100,000 stars over 3+ years, it is collecting a vast repository of stellar data, which can be used to investigate sunspot cycles and flare variability. This is an example of where stellar input data can be used by solar physicists to gain a better perspective of the Sun as a star. Question 2: How do flares on solar-like stars and the Sun compare to flares on extremely magnetically active stars? While observations of stellar flares are not as numerous as those of flares on our well- studied Sun, the general consensus appears to be that stellar flares comprise the same basic physical processes occurring in solar flares. This is despite the fact that the types of stars studied so far can be significantly different from the Sun: F dwarfs (Mullan & Mathioudakis 2000), G and K giants (Ayres et al. 1999, Ayres et al. 2001, Testa et al. 2007), tidally locked RS CVn binary systems (Osten et al. 2004), dMe flare stars (Osten et al. 2005), flares on hyperactive young Suns containing star-disk interactions (Favata et al. 2005), and very low mass stars near the substellar limit (Stelzer et al. 2006). With the advent of sensitive X-ray and radio telescopes, multi-wavelength campaigns on magnetically active stars have revealed many similarities in behavior between solar and stellar flares, which implies that common physical processes unite the phenomena. Yet, because of the observational biases in the types of stars targeted for in-depth study, our understanding of flares and associated effects may be incomplete. Despite the generally good agreement between solar flare models and stellar flare observations, there are still areas of apparent disagreement. M dwarfs have been the subject of flare monitoring due to their propensity for frequent and extreme flaring. The white light and continuum response in a stellar flare on a dMe flare star observationally may take the form of a blackbody with temperatures near 104 K and area coverages ∼0.01% of the stellar disk area (Hawley et al. 2003), with the flare enhancement peaking to the blue end. Radiative hydrodynamic simulations of the response of a stellar flaring atmosphere to the input of a beam of electrons can reproduce other features observed in stellar flares, such as line emission and observed velocity shifts and Stark broadening, but still cannot reproduce the magnitude of the observed continuum enhancements (Allred et al. 2006). This is in contrast to the same kinds of models applied to solar flares, which do show general agreement between observed white-light flares and models (Allred et al. 2005). The solar flare beam heating in an M dwarf stellar atmosphere does not produce the observed continuum increases. The electron beam cannot penetrate deeply into the photosphere to provide direct heating which would result in the observed continuum emission, and backwarming from the X-ray and EUV emission cannot provide the required energy input. The distribution of stellar flares with energy on extremely magnetically active flaring stars appears to be similar to the distribution of large solar flare events (Osten & Brown 1999, Wolk et al. 2005), with evidence for M dwarf flare stars to have a distribution favoring smaller amplitude events (α in the flare frequency-energy distribution ≥2; Güdel et al. 2003). The typical individual large stellar flare represents energetic events at least as powerful as the largest solar flares (and often larger), by virtue of sensitivity thresholds. Power stellar flares many orders of magnitude larger than the largest solar flares have been observed: radiated energies at least 1038 erg, and possibly larger, have been observed on the most hyperactive flare stars (Favata et al. 2005, Osten et al. 2007). Flares whose luminosity at X-ray energies approach or even exceed the starʼs normal radiative output across all wavelengths (Favata et al. 2000, Osten et al. 2007, Osten et al. 2010) have been detected. These observations call out for a description of the maximum flare energy and energy storage in active regions from a theoretical perspective, which the solar community could provide. Interpretation of stellar flares requires the spatial resolution of solar flare data to provide plausible scenarios for what might be happening on flaring stars. The interaction between stellar astronomers and solar physicists in this regard can be a fruitful one, as stellar astronomers can gain from the large solar datasets available, and solar physicists can learn how stellar flares differ from solar flares. Question 3: What is the dependence of flare rate on stellar parameters? Due to their dependence on the presence and dynamics of interacting magnetic fields, stellar flares are a transient diagnostic of magnetic activity, in addition to more commonly used persistent indicators such as chromospheric/coronal emissions, starspots, and rotation. Magnetic activity is a function of convection zone depth and rotation rate, as quantified by the Rossby number (Noyes et al. 1984). This relates ultimately to the dynamo generation of magnetic fields producing the large-scale magnetic fields whose stresses and plasma interactions cause the mechanical heating and reconnection observed as different magnetic activity phenomena. In solar-like stars, magnetic activity is a function of age, with young stars exhibiting magnetic hyperactivity, and a consequent fall-off with increasing age accompanying spin-down. Likewise, the stellar flare rate appears to decline as a function of age. In the young suns of Orion (0.9-1.2 Msun, F7-G5 dwarfs, age ∼106 years), Wolk et al. (2005) estimated X-ray flares more energetic than 1034 erg occurred roughly once per week. This minimum flare energy is more than 100 times more energetic than the largest observed solar flares.
Details
-
File Typepdf
-
Upload Time-
-
Content LanguagesEnglish
-
Upload UserAnonymous/Not logged-in
-
File Pages7 Page
-
File Size-