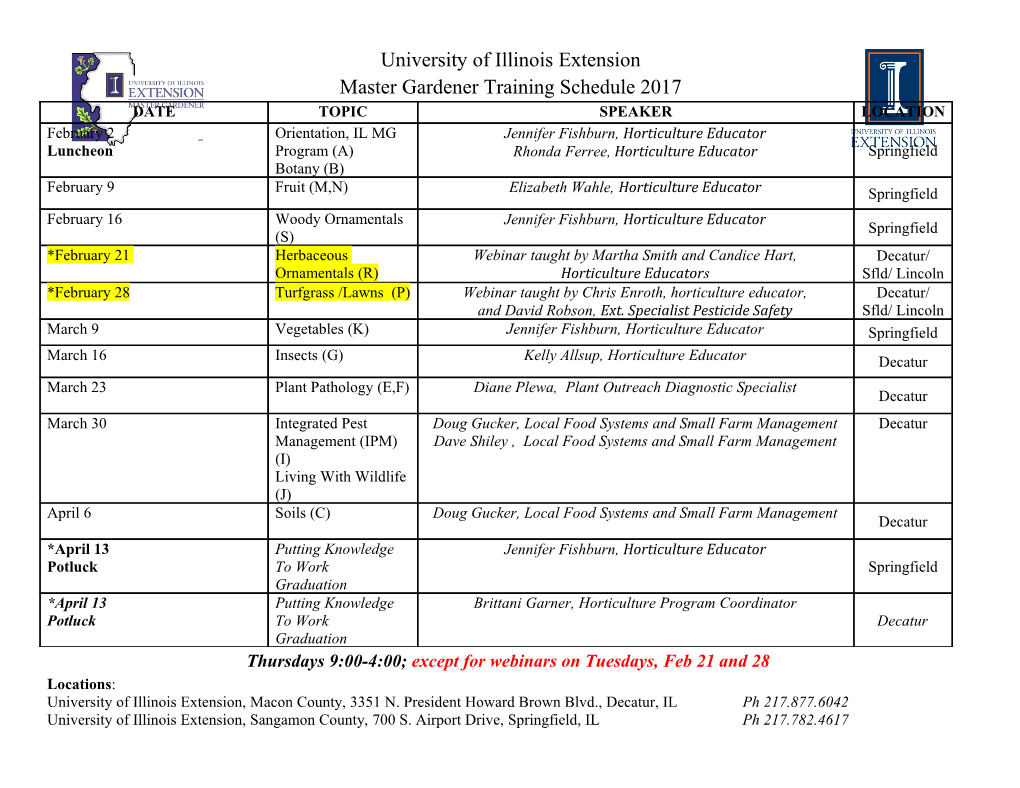
The University of Melbourne School of Mathematics and Statistics PhD Thesis Chevalley Groups and Finite Geometry PhD Student: Jon Yue Zhang Xu (University of Melbourne) Supervisor: Prof. Arun Ram (University of Melbourne) Cosupervisor: A/Prof. John Bamberg (University of Western Australia) May 9, 2018 An austere building cut into simple pieces, now we see clearly. ³WM K;~f Q ikameshiki yawokizamarete satorikeri i Contents i Acknowledgements v Introduction 1 Chapter 1. Finite Geometry 5 1.1. Lattices 5 1.2. Incidence structures 9 1.3. Veblen-Young type theorems 13 1.4. Ovoids of projective incidence structures 14 1.5. Ovoids of polar incidence structures 18 Chapter 2. Chevalley Groups 23 2.1. Lie algebras and root systems 23 2.2. Chevalley bases and Chevalley groups 24 2.3. The Bruhat Decomposition 27 2.4. Decomposing double cosets into single cosets 31 2.5. Examples 36 Chapter 3. Twisted Chevalley groups 49 3.1. Definitions and basic properties 49 3.2. Examples 55 3.3. From sesquilinear forms to Steinberg endormorphisms 64 Chapter 4. Tying things together 67 4.1. (Generalized) flag varieties, their lattices and incidence structures 67 4.2. Ovoids and (twisted) Chevalley groups 71 4.3. The Thickness of Schubert Cells 77 4.4. The example G = SL3(F) 81 Appendix A. 85 A.1. Finite Fields and Galois Theory 85 A.2. Sesquilinear forms 86 A.3. Quadratic forms 89 A.4. Flag varieties and buildings 91 Appendix B. 93 B.1. Hasse diagrams of subspace lattices 93 B.2. Hasse diagrams of Boolean lattices 96 3 B.3. Hasse diagrams of Schubert cells in PG(F2) 99 Bibliography 103 Index 109 iii Acknowledgements I would like to thank my supervisor Arun Ram for his honesty and determination in the PhD supervision process. He has been an inspiring figure, and he has taught me how to confront mathematics seriously and professionally. I would like to thank my cosupervisor John Bamberg for helping me learn finite geometry, and for his hospitality during my six month visit at the University of Western Australia. The haiku in the opening page of the thesis was submitted to the University of Melbourne's Graduate Students Association thesis haiku competition, where it won (joint) first place. I would like to thank Asako Saito and Haydn Trowell for help with the Japanese translation. v Introduction The aim of this thesis is to show how aspects of representation theory can be used to study finite geometry, and how the finite geometric concept of `thickness' can be used to study Schu- bert cells (from representation theory). It is a result of an effort to create \interdisciplinary" communication and collaboration between the finite geometry community and the represen- tation theory communities in Australia, and we hope that this thesis can help to bridge the modern language barrier between these two fields. The books of D. Taylor [Tay92], Z. Wan [Wan93], Buekenhout and Cohen [BC13], are already important contributions to this dialogue. We chose the finite geometry question of finding and classifying ovoids as a framework for investigation. The goal was to shape the language of algebraic groups and Chevalley groups to provide tools for studying ovoids. The precedent in the work of Tits [Tit61] and Steinberg [Ste67, Example (c) before Theorem 34] on the Suzuki-Tits ovoid indicated that this was a fruitful research direction. Chapter 1, Chapter 2 and Chapter 3 cover well-known definitions and results from (respec- tively) finite geometry, the theory of Chevalley groups, and the theory of twisted Chevalley groups. Most of the theory and examples have been adapted from the literature. The following parts do not explicitly appear in the literature, and may be useful to those interested in aspects of Chevalley groups and/or finite geometry: • the proof that the classical ovoid in the Hermitian space H(3; q) is an ovoid (Proposition 1.5.5), • the worked examples after Theorem 2.4.6 of the indexing of the points of the flag variety G=B, • the worked examples of twisted Chevalley groups in Chapter 3, • the lattice theory discussed in Section 1.1.4 used to produce the pictures of Schubert cells in Section B.3 (these pictures provide a novel way to view Schubert cells and flag varieties). Chapter 4 constitutes our main contribution to the research literature, which consists of three theorems: Main Theorem 1. Let C be the favourite nondegenerate conic, let E be the favourite elliptic quadric, and S the favourite Suzuki-Tits ovoid as defined in (respectively) Equation 1.1, Equation 1.2, Equation 1.3. ∼ (1) (Section 4.2.1) Let G = P Ω3(Fq) (= Ω3(Fq)=Z(Ω3(Fq), where Ω3(Fq) is the commuta- tor subgroup of O3(Fq)) and B be the subgroup of upper triangular matrices. With G 2 2+1 + 2 acting on P = P(Fq ) by matrix multiplication and [v ] = [1 : 0 : 0] 2 P , then Φ: G=B −! 2 P is injective with Φ(G=B) = C: gB 7−! g[v+] F − F (2) (Section 4.2.2) Let G = P Ω4 (Fq) be the orthogonal group of minus type and B be F 3 3+1 the subgroup of upper triangular matrices. With G acting on P = P(Fq ) by matrix multiplication and [v+] = [1 : 0 : 0 : 0] 2 P3, then Φ: GF =BF −! 3 P is injective with Φ(GF =BF ) = E: gBF 7−! g[v+] 1 F F F (3) (Section 4.2.3) Let G = Sp4(F22e+1 ) be the Suzuki group and B be the subgroup of F 3 3+1 upper triangular matrices. With G acting on P = P(Fq ) by matrix multiplication and [v+] = [1 : 0 : 0 : 0] 2 P3, then Φ: GF =BF −! 3 P is injective with Φ(GF =BF ) = S: gBF 7−! g[v+] Main Theorem 2. (Section 4.2.4) Let O be the favourite classical ovoid in the Hermitian 3+1 space H(Fq2 ) as defined in Proposition 1.5.5. This means that O is the set of totally isotropic 1-dimensional subspaces in U ?, where U = [x−2 : x−1 : x1 : x2] is a choice of nondegenerate 1-dimensional subspace. Then O has a Schubert cell decomposition given by q O = f[1 : 0 : 0 : 0] j x2 = 0g t f[u : 1 : 0 : 0] j u 2 Fq2 and x2u − x1 = 0g q t f[u : t : 1 : 0] j u 2 Fq2 ; t 2 Fq and x2u − x1t + x−1 = 0g 0q 0q q 0 0 q 0q t f[t − u u : −u : u : 1] j u; u 2 Fq2 ; t 2 Fq and x2(t − u u ) + x1u + x−1u − x−2 = 0g t f[t0 − u0qu : −u0q + tuq : uq : 1] 0 0 0 0 q 0 j u; u 2 Fq2 ; t; t 2 Fq and x2(t − u u ) − x1(−u + tu) + x−1u − x−2 = 0g: Main Theorem 3. (Section 4.3) Let G(Fq) be a Chevalley group and let Pi and Pj be the ith and jth standard maximal parabolic subgroups of G(Fq). Let W be the Weyl group of ˚ G(Fq), w 2 W and let Xw = BwB be the Schubert cell corresponding to w. Then the number ˚ of elements in BwPi incident to gPj in Xw is `(z) j i;j q ; where w = uzv with u 2 W , zv 2 Wj, z 2 (Wj) , v 2 Wi;j. We now more informally describe the results, motivations and methodology of these theo- rems. In Section 4.1.4, we establish a relationship between incidence structures (from finite ge- ometry), and flag varieties (from representation theory). The motivation for establishing this relationship is the work of Tits and Steinberg on the Suzuki-Tits ovoid, and is outlined in Section 4.2.3. Main Theorem 1 explicitly describes three key examples of ovoids { the rational normal curve, the elliptic quadric, and the Suzuki-Tits ovoid { as flag varieties of a suitably chosen Chevalley group. It would be interesting to consider whether non-classical ovoids (see [Che04] and [Che96]) can also be realised in this way, with perhaps the role of the Chevalley group being played by a suitably chosen pseudo-reductive group (see [CGP15]). Main Theorem 2 provides a Schubert cell decomposition of the classical ovoid in the Her- mitian variety H(3; q2) (as described in Proposition 1.5.5). Before describing Main Theorem 3, let us review the definitions of ovoids (in finite geometry) and Schubert cells (in representation theory). Ovoids. Let V be a vector space and let PG(V ) be the lattice of subspaces of V with inclusion ⊆ as the partial order. A point (respectively line, hyperplane) in PG(V ) is a subspace S ⊆ V such that dim(S) = 1 (respectively dim(S) = 2; dim(S) = dim(V ) − 1). Let O be a set of points in PG(V ). A tangent line to O is a line in PG(V ) that contains exactly 1 point of O. Then [Tit62, §1] defines, an ovoid of PG(V ) as a set O of points of PG(V ) such that (O1) (thinness) If l is a line in PG(V ) then l contains 0, 1 or 2 points of O, (O2) (maximality) If p 2 O then the union of the tangent lines to O through p is a hyper- plane. These two types of conditions, \thinness" and \maximality", characterize the definitions of ovoids (and ovals and hyperovals) in projective spaces, projective planes, polar spaces and 2 generalized quadrangles that can be found in the finite geometry literature (see, for example, [HT15], [Bal15, §4.8], [Bro00a, §1] and [BW11, §2.1 and §4.2 and §4.4]).
Details
-
File Typepdf
-
Upload Time-
-
Content LanguagesEnglish
-
Upload UserAnonymous/Not logged-in
-
File Pages118 Page
-
File Size-