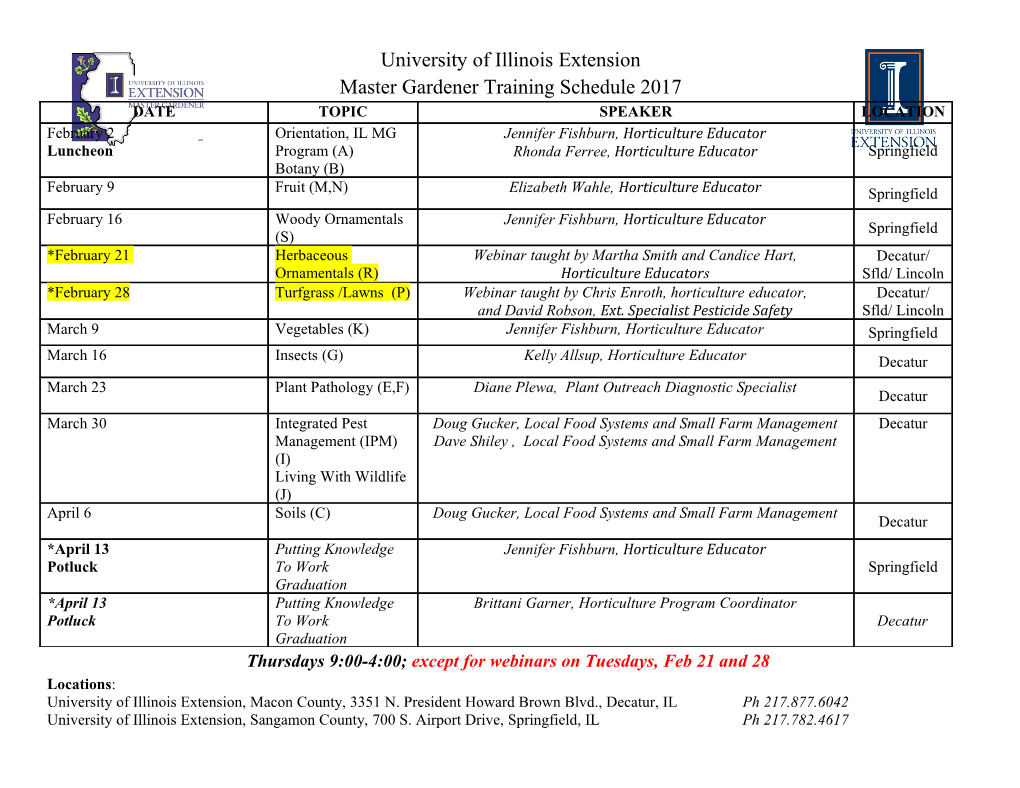
Genome-wide screen reveals novel mechanisms for sodium dodecyl sulfate toxicity in Saccharomyces cerevisiae Chunlei Cao Jiangnan University Zhengfeng Cao Yangzhou University Peibin Yu Jiangnan University Yunying Zhao ( [email protected] ) Jiangnan University Research article Keywords: Budding yeast, SDS, Genetic screening, Genomics, ROS Posted Date: January 2nd, 2020 DOI: https://doi.org/10.21203/rs.2.15488/v2 License: This work is licensed under a Creative Commons Attribution 4.0 International License. Read Full License Page 1/20 Abstract Background: Sodium dodecyl sulfate (SDS) is one of the most widely used anionic alkyl sulfate surfactants. Toxicological information on SDS is accumulating, however, mechanisms of SDS toxicity regulation remain poorly understood. In this study, the relationship between the SDS-sensitive mutants and their intracellular ROS levels has been investigated. Results: Through a genome-scale screen, we have identied 108 yeast single-gene deletion mutants that are sensitive to 0.03% SDS. These genes were predominantly related to the cellular processes of metabolism, cell cycle and DNA processing, cellular transport, transport facilities and transport routes, transcription and the protein with binding function or cofactor requirement (structural or catalytic). Measurement of the intracellular ROS (reactive oxygen species) levels of these SDS-sensitive mutants showed that about 79% of SDS-sensitive mutants accumulated signicantly higher intracellular ROS levels than the wild-type cells under SDS stress. Moreover, SDS could generate oxidative damage and up-regulate several antioxidant defenses genes, and some of the SDS-sensitive genes were involved in this process. Conclusion: This study provides insight on yeast genes involved in SDS tolerance and the elevated intracellular ROS caused by SDS stress. Our ndings provide a basis to understand molecular mechanisms underlying the detoxication of SDS by yeast cells. Background Surfactants are organic pollutants distributed widely in the current environment, and their toxicity has caused widespread concern. One of the synthetic anionic surfactants, sodium dodecyl sulfate (SDS), or sodium lauryl sulfate (SLS), a product that consists of approximately 70 % sodium dodecyl sulfate and 30 % sodium tetradecyl sulfate, with the formula of CH3(CH2)11OSO3Na, has been used in many cleaning and hygiene products such as liquid soaps, shampoos, bubble baths, shower gels, and nearly all toothpastes. SDS is also used in pharmaceutical and food products, as well as in industiral and laboratory applications, i.e, SDS can form complexes with protein through hydrophobic interactions and thus be used in polyacrylamide gel electrophoresis to determine the molecular weight of proteins [1, 2]. The concentration of SDS found in consumer products varies by product and manufacturer but typically ranges from 0.01% to 50% in cosmetic products and 1% to 30% in cleaning products [3]. The lethal dose, 50% (LD50) for SDS is 0.8–1.10 g/kg in rats, SDS concentrations 2% are considered irritating to normal skin in human patch testing, and >5% causes depression, labored breathing, diarrhea, and death ( four out of 20 animals) [2]. Safety concerns with SDS application in human include carcinogenicity, skin and eye irritation, and aphthous ulcers. The toxicity of SDS has been demonstrated in bacteria, microalgae, crustaceans, echinoderms, rats, humans and carp. The basis of SDS toxicity seems to be mainly related to the alteration of the cellular ionic balance caused by cellular membrane permeability alterations and to the induction of oxidative stress, that can generate other physiological and biochemical stresses [4]. SDS elicits both physical and biochemical effects on cells, with the membrane the primary target structure, and considered as a a typical cell wall perturbing agent. Effects are concentration dependent and range from loss of barrier function and increased permeability to complete cell lysis. It is suggested that SDS causes elevated the glutathione production, lipid peroxidation as well as changes in carbon metabolism [5], leading to altered cell membrane stability and permeability as well as indirectly to increased accessibility of cell wall [6]. Yeast cell wall serves crucial functions in protecting against osmotic shock stress and mechanical steess, maintaining cell shape, as well as serving a sacffold for cell-surface proteins [7]. SDS interrupts cell membranes and then triggers the Cell Wall Integrity (CWI) signaling pathway, a kinase cascade to maintain cell Page 2/20 integrity and can be activated by chemicals that damage the cell wall and membrane in buding yeast [8]. For example, the Slt2/Mpk1, a mitogen-activated protein (MAP) kinase, can be phosphorylated and thus activated by impaired cell integrity [9]. However, deatiled mechanisms of SDS toxicity in microorganisms or higher eukaryotes are poorly understood. Yeast has been previously used to demonstrate the effect of SDS on biological membranes, showing that micelles of SDS may penetrate the membrane through pores in the yeast cell wall and destroy the membrane [10]. In defense against SDS surplus, yeast cells increase the expression levels of genes involved in oxidative stress which might be caused by its effect on membrane structure, carbon metabolism, or DNA repair [2]. Reactive oxygen spesies (ROS) play an important role in inducing cell death or apotosis in yeast cells by causing damages to proteins, lipids and DNA [11, 12]. In addtion, ROS could induce cell wall damage in yeast cells lacking mitochondrial DNA, making cells to become more sensitive to of SDS stress [13]. As the simplest eukaryotic organism, the budding yeast Saccharomyces cerevisiae (S. cerevisiae) has been used to identify the mechanism and regulation of metal ion transport [14]. Here, we used S. cerevisiae to explore the SDS effect on eukaryotic cell growth and compared the oxidative stress (reactive oxygen species, ROS) in cultured cells. We have rstly screened the SDS-sensitive mutants from the yeast nonessential gene deletion library and identied 108 SDS-sensitive mutants. To evaluate whether SDS generates serious oxidative stress to the SDS-sensitive mutant cells, we have then measured the cellular response of cultured yeast cells to SDS in terms of ROS levels. Specically, we show that SDS can induce oxidative stress and that yeast cells eliminate these oxidative damage by elevating the expression levels of the genes coding for antioxidant defenses. Results An overview of genes involved in the SDS sensitivity of yeast cells To investigate the cellular functions required for cell growth under a surplus of SDS, a yeast library of diploid nonessential gene deletion was screened to identify genes involved in the sensitivity to SDS. The results show that 108 gene deletion mutants (2.3% of the screened 4757 gene deletion mutants) were identied as sensitive to 0.03% SDS (Fig. 1 and Table 1). The genotypes of these 108 mutants were conrmed by PCR with the forward primer derived from the promoter region of each correspondent gene and a reverse primer KanMX4-R (Additional le 1: Table S1 and Additional le 2: Figure S1) derived from the ORF region of KanMX4. The functional categories of these 108 genes are involved in metabolism (17), cell cycle and DNA processing (15), transcription (14), cellular transport, transport facilities and transport routes (28), biogenesis of cellular components (6), cellular communication / signal transduction mechanism (2), protein with binding function or cofactor requirement (structural or catalytic) (10), as well as unclassied proteins (16) (Table 1). Gene Ontology (GO) enrichment analysis result showed that these 108 SDS-sensitive genes were mainly enriched in vacuolar transport, ATP export, and endosomal transport among the top 16 GO terms in cluster groups (Additional le 3: Figure S2). Exposure to SDS stress results in ROS generation Since SDS had been conrmed to induce the oxidative stress response [2], we next measured the intracellular ROS levels of the 108 SDS-sensitive mutants under 0.015% SDS treatment. In the wild-type BY4743 cells, the intracellular Page 3/20 ROS level was signicantly increased under SDS stress (Fig. 2 and Additional le 4: Figure S3). Interestingly, only six mutants for ARG82, TRP5, GRR1, MSH1, LAS21, and YNL296W of these 108 SDS-sensitive mutants, accumulated lower intracellular ROS levels when treated with 0.015% SDS than without SDS (The relative ROS levels in these mutants was smaller than 1; Fig. 2 and Additional le 4: Figure S3). It suggested that the above six genes might not be directly involved in the regulation of intracellular ROS levels under SDS stress. Of these 108 SDS-sensitive mutants, 85 mutants accumulated signicantly higher intracellular ROS levels under SDS stress compared with wild-type cells (Additional le 4: Figure S3B and D), indicating that these 85 mutants might respond to lower concentration of SDS and thereby accumulated higher ROS levels than wild type cells. The rest 23 mutants accumulated similar or lower intracellular ROS levels when treated with SDS compared with wild type cells, although the relative ROS levels in some of these mutants were also very high (Fig.2 and Additional le 4: Figure S3B and D). Here we showed that mutants for genes related to the functions of metabolism and cellular transport, transport facilities and transport routes were most sensitive to SDS stress (Table 1). We listed some genes as the representative genes of their
Details
-
File Typepdf
-
Upload Time-
-
Content LanguagesEnglish
-
Upload UserAnonymous/Not logged-in
-
File Pages20 Page
-
File Size-