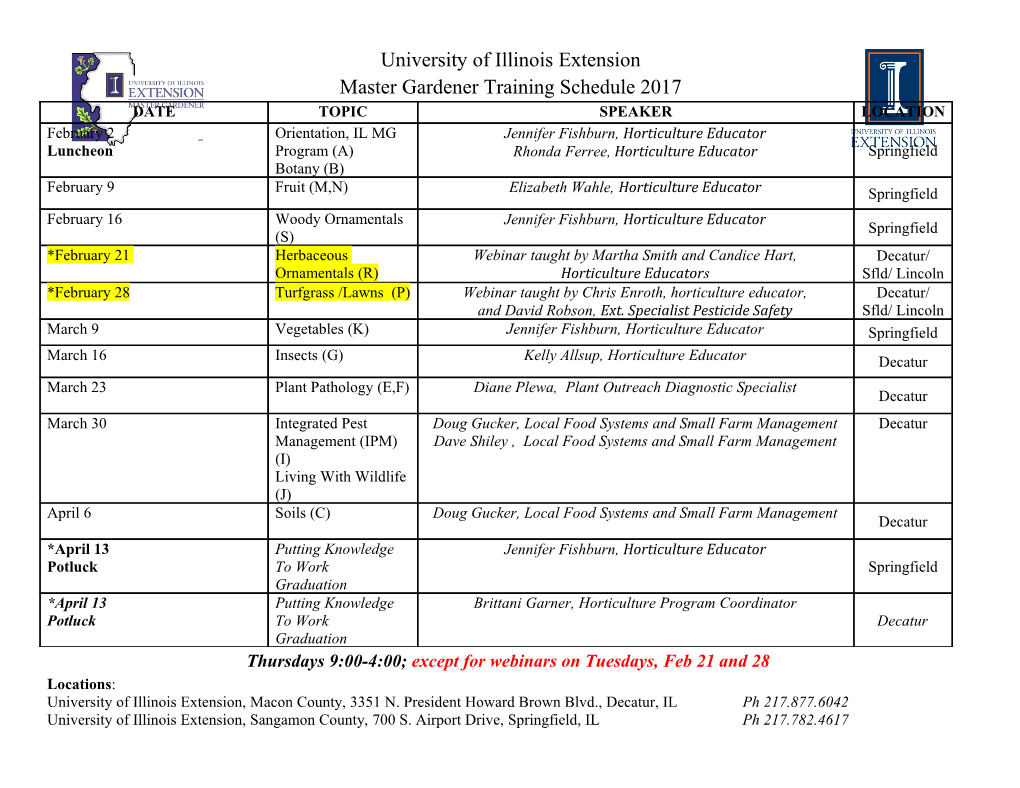
Flow Control of Compressible Dynamic Stall using Vortex Generator Jets THESIS Presented in Partial Fulfillment of the Requirements for the Degree Master of Science in the Graduate School of The Ohio State University By Shawn Christopher Naigle Graduate Program in Aeronautical and Astronautical Engineering The Ohio State University 2016 Master's Examination Committee: Professor Jeffrey P. Bons, Advisor Professor James W. Gregory Copyright by Shawn Christopher Naigle 2016 Abstract Dynamic stall is an airspeed and maneuver limiting event which occurs on helicopter retreating blades at high advance ratios and is associated with aerodynamic flutter and large negative pitch moments. The potentially violent dynamic stall sequence amplifies pitch link stress and can lead to loss of aircraft control. Steady Vortex Generator Jet (VGJ) blowing has proven to delay the onset of dynamic stall. This work presents results of an experimental investigation into active flow control of a Sikorsky SSC−A09 airfoil undergoing periodic pitching motion in a variety of flow conditions including, steady incompressible, steady compressible, and time-varying compressible freestream, representative of a helicopter rotor system in flight. The airfoil was evaluated at reduced pitching frequencies of 0.025≤ k≤0.050 with a nominal angle of attack schedule, α=9.5°−10.5°cos(ωt). Flow conditions were at steady Mach numbers of M=0.2 and M=0.4 and time-varying phase-locked freestream oscillations at Mach number M=0.4+0.07cos(ωt), at Reynolds numbers 1.5×106≤ Re≤3.0×106. Flow control was achieved through a spanwise row of jets located at 10% chord, oriented normal to the surface, with an effective activated control width of 75% airfoil span. Blowing flow control was evaluated at a jet mass flux ratio 0.002≤ Cq≤0.005. Flow control enhancements evaluated include stall penetration, lift and moment improvements, reduction in negative damping, and flow reattachment angle. Quantitative ii measurements of lift and moment coefficients were calculated through the integration of airfoil surface pressure taps. Qualitative, time-resolved Background Oriented Schlieren (BOS) supplemented surface pressure measurements to assess spanwise averaged dynamic stall vortex progression as well as shock interaction. No optimal mass flux ratio completely controls dynamic stall, but VGJs delayed boundary layer separation, consistently improved cycle average moment, and increased cycle average lift. VGJs triggered an earlier flow reattachment which reduced hysteresis and circuits of clockwise rotation on the CM curve related to negative damping. BOS imagery confirmed the presence of leading edge shock formation and showed VGJ capability to delay shock-induced flow separation. The effectiveness of VGJ flow control is primarily a function of maximum angle of attack, pitching frequency, and freestream compressibility. A comparison of VGJ flow control evaluated on a pitching airfoil in a steady compressible freestream at M=0.4 versus a pitching airfoil in a time-varying compressible freestream at M=0.04+0.07cos(ωt) at matched mean reduced frequency and Reynolds number, experienced similar quantitative improvements. Comparison of BOS imagery reveal the same physical VGJ to shear layer interaction between the steady and time- varying freestream cases. Thus, performance measurements based on active VGJs in a steady compressible freestream provide a good prediction of the expected performance measurements when blowing is applied to an airfoil in a low amplitude, time-varying compressible freestream. At low freestream oscillations, airfoil pitching frequency is the dominant factor influencing VGJ effectiveness. iii Dedication To my loving wife, Laura, beautiful daughter Vera, and fellow students. iv Acknowledgments This thesis would not have been completed without the assistance and support from all of my co-workers at The Ohio State University Aerospace Research Center. To my fellow students, thank you for your tutelage on the systems, software, and general concepts; especially Matthew Frankhouser, Kyle Hird, Kevin Disotell, and Kevin Williams. Your extra efforts were invaluable. A special thank you to Josh Gueth for assistance in component manufacturing and ensuring resources were always available for completion of this study. I would be remiss if I did not recognize the critical professional guidance, patience, and mentorship from Dr. James Gregory and my advisor, Dr. Jeffrey Bons. Thank you both for challenging me and helping me grow throughout the entire graduate school experience. Thank you to the Department of the Army for not only offering me the opportunity to attend The Ohio State University, but also to the Army Research Office for funding this investigation. Most importantly, thank you to my family, especially my wife, Laura. From the late-night dinners for study groups at the lab to re-teaching me calculus, your unwavering support did not go unnoticed and this thesis is as much yours as it is mine. Lastly, to my little girl, Vera, thank you for being an unrelenting, but wonderful distraction – I love my ladies. v Vita 2000 ............................................................ Diploma, Centreville High School 2005 ........................................................... B.S. Mechanical Engineering, United States Military Academy Fields of Study Major Field: Aeronautical and Astronautical Engineering vi Table of Contents Abstract .......................................................................................................................... ii Dedication ..................................................................................................................... iv Acknowledgments ...........................................................................................................v Vita ............................................................................................................................... vi List of Tables................................................................................................................. ix List of Figures .................................................................................................................x Nomenclature ...............................................................................................................xvi Chapter 1: Introduction ....................................................................................................1 Section 1.1: Rotorcraft Mechanics and Dynamic Stall ..................................................2 Section 1.2: Flow Control of Dynamic Stall ............................................................... 13 Section 1.3: Normal Oriented Vortex Generator Jets Flow Control ............................. 17 Chapter 2: Experimental Methodology .......................................................................... 23 Section 2.1: Experimental Facility .............................................................................. 23 Section 2.2: Data Acquisition ..................................................................................... 29 Section 2.3: Test Article ............................................................................................. 31 Section 2.4: Tunnel Instrumentation ........................................................................... 34 vii Section 2.5: Dynamic Compensation .......................................................................... 36 Section 2.6: Vortex Generator Jets ............................................................................. 37 Section 2.7: Background Oriented Schlieren .............................................................. 40 Section 2.8: Measurement Uncertainties ..................................................................... 44 Chapter 3: Results and Discussion ................................................................................. 46 Section 3.1: Static Airfoil in a Steady Freestream ....................................................... 48 Section 3.2: Airfoil Pitch Oscillations in a Steady Freestream .................................... 58 Section 3.2.1: Airfoil Pitch Oscillations in an Incompressible Freestream ............... 59 Section 3.2.2: Airfoil Pitch Oscillations in a Compressible Freestream ................... 69 Section 3.3: Airfoil Pitch Oscillation in a Time-Varying Compressible Freestream .... 86 Section 3.4: Time-Resolved Background Oriented Schlieren .................................... 102 Chapter 4: Conclusion ................................................................................................. 119 References ................................................................................................................... 123 Appendix A: Dynamic Compensation Modeling .......................................................... 128 Appendix B: Non-Optimal Results .............................................................................. 133 viii List of Tables Table 1: Bergh-Tijeman tubing and sensor geometry. .................................................. 128 Table 2: Amplification and phase delay of airfoil model. ............................................. 132 ix List of Figures Figure 1: Schematic of rotorblade relative velocity in forward flight [2]. .........................3 Figure 2: Diagram of rotor disc lift regions in forward flight [2]. .....................................3 Figure 3: Hypothetical rotorcraft pitch angle in high speed forward flight [2]. .................4
Details
-
File Typepdf
-
Upload Time-
-
Content LanguagesEnglish
-
Upload UserAnonymous/Not logged-in
-
File Pages155 Page
-
File Size-