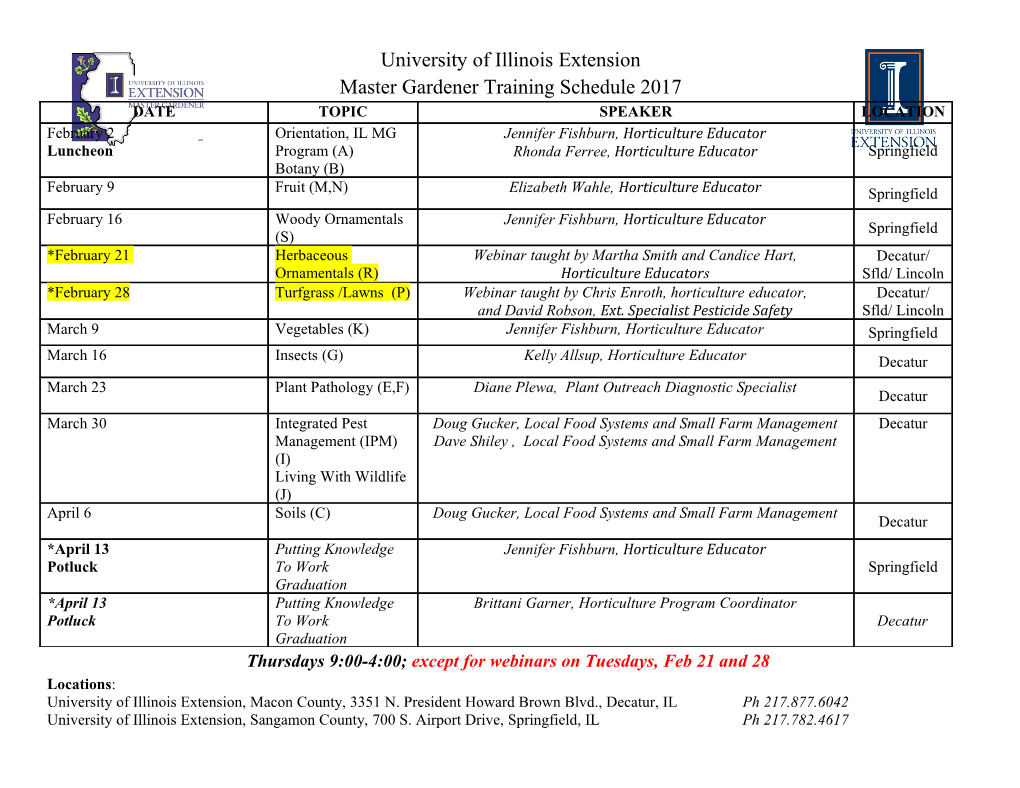
10: Twinkle, Twinkle Little Star Physics 17: Black Holes and Extreme Astrophysics Stars • The Sun is a main sequence star, powered by nuclear fusion reactions (H → He) in the core • Stars form when a cloud of gas collapses under the force of gravity (the mutual attraction of every part of the cloud to every other part) • Nuclear fusion begins when gravity compresses the core to high enough density, pressure & temperature • When hydrogen fuel runs out in the core, the core collapses under gravity, heats the envelope, which expands, forming a red giant • High mass stars form red supergiants. High enough pressures reached to fuse elements beyond hydrogen • Elements up to iron are formed by nuclear fusion in red supergiants Goals • Understand what happens when stars come to the ends of their lives • See how quantum mechanics can save lower mass stars from ultimate collapse • Introduce neutron stars and pulsars • Find out how stars collapse into black holes Reading Begelman & Rees • Chapter 2 • Stars (p24-41) • Neutron stars and black hole formation (p41-49) Butterfly Nebula Nebulae • As stars come to the ends of their lives, gas pressure and radiation pressure (Lecture 7) from the hot, collapsing core can cause the outer layers of the star to be ejected in a wind Dumbbell Nebula • Can form a planetary nebula Cat’s Eye Nebula • In a Sun-like star, the core stops collapsing and forms a white dwarf, inside the nebula Ring Nebula Supernovae SN 1987A Cassiopeia A • For stars > 8�⨀ • After fusion reactions all the way to iron, star can no longer extract nuclear energy in fusion reactions — there is no longer a source to heat the gas and resist the pull of gravity • Star collapses extremely rapidly (final Crab Nebula burning takes ~1 day, collapse in seconds) • Enormous release of gravitational energy • Blows outer layers of star away — leaves behind expanding remnant of gas • One star can outshine the whole galaxy when it explodes Supernova in NGC 4526 Supernovae are an important part of Energy release in a supernova the galactic ecosystem 1044 J kinetic energy per solar mass of material, travelling at 10,000 km s-1. It • As the star collapses, ~1046 J energy is released travels ~10 ly before it decelerates: • Is the core gets compressed, electrons from the edge of atoms combine with protons in the nucleus, forming neutrons in core • Heats interstellar gas ! � + � → � + �" • Make new stars (the kinetic energy • This beta process releases neutrinos — collapsing gas is so dense, pushes the interstellar gas and can photons cannot escape, but weakly interacting neutrinos can — stimulate it to collapse) much on the energy is released in neutrinos • Disseminate heavy elements that are • Neutrinos transfer kinetic energy into the collapsing envelope of made in nuclear reactions in the star the star — drives out the infalling gas at 10,000 km s-1, which is — the material the Sun, Earth and 44 10 J kinetic energy per solar mass of ejected material — this is the Solar System are made of must have explosion once been through a previous • A shock wave heats the gas and makes it shine with peak power generation of stars and then scattered 1036 W for ~10 days. This is 1042 J in luminosity (outshines galaxy) in a supernova explosion BigI BigI What happens next??? • The fate of the star depends upon how much mass remains in the core that’s left behind after the supernova explosion (high mass stars) the nebula is blown off (low mass stars) • Massive stars (~20�⊙) can lose up to 40% of their mass in winds during the ref supergiant phase • Several Solar masses can be blown off in the supernova • The gravitational force pulling the core together gets stronger, so the core will keep collapsing, unless another force can balance gravity WILtY Particle-wave duality Quantum Mechanics in 1 slide • Light can be described as either a wave or as particles (photons) (as well as the high frequency wave, The (squared) amplitude • Particles can also be described as a low frequency component is the probability of describes the localization) finding the particle at waves — the wavefunction of the each position particle A particle no longer has a location, but a probability of finding it in a given place The wavelength/frequency (and, likewise, a probability of it being represents the momentum found with a particular momentum) Heisenberg’s Uncertainty Principle: We cannot simultaneously and Quantum mechanical effects become precisely know both a particle’s position and momentum (because if important on small scales (~atoms) the wavefunction above is localized to one position and is zero everywhere else, we cannot define its wavelength/momentum) While it seems strange, quantum ℏ mechanics is a well-tested physical ∆� ∆� ≥ 2 theory that physicists have complete ! $%& ℏ = ⁄"#, where Planck’s constant ℎ = 6.63×10 J s faith in The Chandrasekhar mass limit White Dwarfs • As the electrons are confined to smaller spaces, their momenta increase • As the remnant of a star collapses, each electron (in the atoms) is confined to a smaller space — ∆� gets small, so ∆�, the uncertainty in the • Eventually they will become relativistic, � = momentum gets large (� = �� — Lecture 3) ���" becomes important, and they cannot • a single frequency wave is not localized, the more confined the electron, the move faster than the speed of light more frequency components (momentum states) we need in the wavefunction • The supporting force provided by the • The Pauli Exclusion Principle: no two fermions (electrons, protons…) may degeneracy pressure of the electrons reaches occupy the same quantum state a limit • As the star collapses, to obey the exclusion principle, all each electron must • If the mass of the stellar remnant is greater occupy a different state, starting at the lowest energy state, up to the Fermi energy than the Chandrasekhar Mass Limit, electron degeneracy pressure cannot contend with • Some of the electrons must occupy high energy states. They have high gravity, a white dwarf would be unstable, and WILtY momentum and high kinetic energy. We must do work to give the electrons this energy, so as we try to compress the electrons, they push back (degeneracy the star continues to collapse pressure) • As the star collapses, the degeneracy pressure eventually equals the A low mass star will collapse into a gravitational pressure collapsing the star. This quantum mechanical force white dwarf, supported by quantum saves the star from further collapse, and forms a white dwarf mechanical forces from the electrons if • Stable stellar remnant, heated by the collapse, gradually cools over time � < 1.46�⊙ BigI Neutron Stars for stars that produced a core-collapse supernova • As massive stars collapsed, electrons in atoms combined with protons, leaving behind a core of neutrons — a neutron star • Neutrons must also obey the Pauli exclusion principle, so when they are compressed, they exert pressure • Mass ~2�⊙ • Radius ~10 km, 3 times bigger than black hole • Neutrons are more massive than electrons, so exert a larger "# $% degeneracy pressure than electrons • Core density ~10 kg m The mass of a star, compressed into a volume the size • Can support a stellar remnant up to 2~3�⊙ of a city! • The exact mass limit is not known because we do not fully understand the structure of the core, which has similar density to A high mass star will explode as a an atomic nucleus — the forefront of nuclear physics supernova, then collapse into a neutron • Likely the core is not composed of individual neutrons — star if the remaining mass in the core neutrons interact with one another via the strong nuclear force � < 2~3�⊙ BigI BigI Pulse observed when beam Pulsars Rotation passes Neutron stars can support strong magnetic fields • As the neutron star spins, magnetic spins with it • Moving magnetic fields create a voltage (electrical Jocelyn Bell-Burnell and Tony Hewish (1967, generator) Cambridge UK) discovered sources of radio • Electrons ripped from the waves emitting regular pulses, seconds apart surface and accelerated, producing radio waves (and Radiation from Jokingly named LGM-1 (‘little green men’) gamma rays) magnetic poles Quickly associated with spinning neutron stars Radio waves are beamed along the axis of the magnetic (predicted by Zwicky & Baade soon after the field – we see the pulse when the beam from the spinning discovery of the neutron in the 1930s) star crosses our line of sight, like a lighthouse WILtY Neutron stars are extreme laboratories! • Matter at extremely high (nuclear) densities • Strong magnetic field (108~1015 x Earth’s field) • Superconducting — electrical currents flow forever • Superfluid — no viscosity • Strong gravity at surface • Gravitational redshift of light emitted • Escape velocity ~0.5c • Relativity is important on a neutron star! BigI The Final Collapse If the mass of the core that remains after the supernova explosion exceeds ~3�⊙, no force is able to counteract the gravitational collapse of the star. The stellar remnant continues to collapse, until it becomes smaller than the Schwarzschild radius for its mass, � 2�� � = �# 6 �7 The remnant disappears inside its event horizon and forms a black hole The material inside the event horizon continues to collapse. Reaches extreme densities (more dense than an atomic nucleus) which will require quantum gravity to describe – the singularity BigI ≲ 2�⨀ left White Dwarf behind Low Mass Star Red Giant Planetary Nebula Neutron Star Giant Star ≳ 8�⨀ Red Supergiant Supernova Explosion Black Hole ≳ 2�⨀ left behind The Crab
Details
-
File Typepdf
-
Upload Time-
-
Content LanguagesEnglish
-
Upload UserAnonymous/Not logged-in
-
File Pages22 Page
-
File Size-