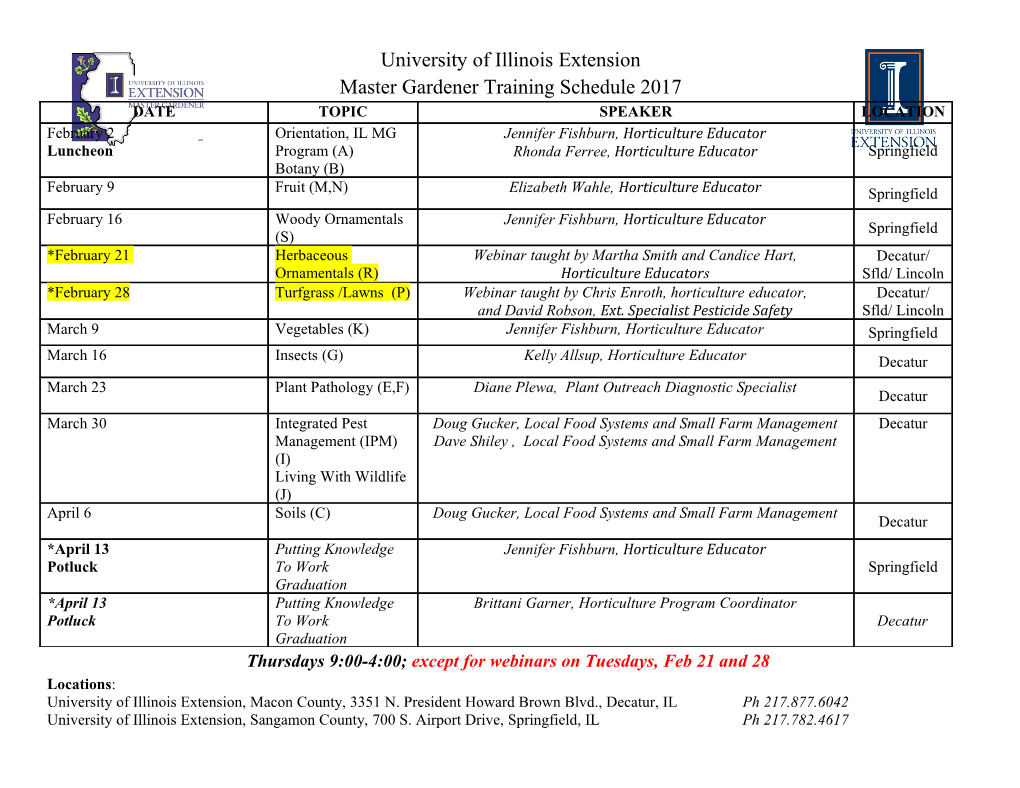
Global and Planetary Change 144 (2016) 213–227 Contents lists available at ScienceDirect Global and Planetary Change journal homepage: www.elsevier.com/locate/gloplacha Invited review article Lake Vanda: A sentinel for climate change in the McMurdo Sound Region of Antarctica Devin N. Castendyk a,⁎,MaciejK.Obrykb, Sasha Z. Leidman c, Michael Gooseff d, Ian Hawes e a Hatch, 143 Union Blvd, Suite 1000, Lakewood, CO 80228, USA b Department of Geology, Portland State University, 1721 SW Broadway, Portland, OR 97201, USA c Department of Earth and Planetary Sciences, University of California, Davis, One Shields Ave., Davis, CA 95616, USA d Institute of Arctic and Alpine Research, University of Colorado, Boulder, 1560 30th Street, Boulder, CO 80303, USA e Gateway Antarctica, University of Canterbury, Ilam Road, Christchurch 8140, New Zealand article info abstract Article history: Lake Vanda is a perennially ice-covered, meromictic, endorheic lake located in the McMurdo Dry Valleys of Ant- Received 29 August 2015 arctica, and an exceptional sentinel of climate change within the region. Lake levels rose 15 m over the past Received in revised form 9 May 2016 68 years in response to climate-driven variability in ice-cover sublimation, meltwater production, and annual dis- Accepted 13 June 2016 charge of the Onyx River, the main source of water to the lake. Evidence from a new bathymetric map and water Available online 22 July 2016 balance model combined with annual growth laminations in benthic mats suggest that the most recent filling trend began abruptly 80 years ago, in the early 1930s. This change increased lake volume by N50%, triggered Keywords: Lake level rise the formation of a new, upper, thermohaline convection cell, and cooled the lower convection cell by at least Thermohaline convection 2 °C and the bottom-most waters by at N4 °C. Additionally, the depth of the deep chlorophyll a maximum rose Limnology by N2 m, and deep-growing benthic algal mats declined while shallow benthic mats colonized freshly inundated Ecology areas. We attribute changes in hydrology to regional variations in air flow related to the strength and position of Bathymetry the Amundsen Sea Low (ASL) pressure system which have increased the frequency of down-valley, föhn winds McMurdo Dry Valleys associated with surface air temperature warming in the McMurdo Dry Valleys. The ASL has also been implicated Wright Valley in the recent warming of the Antarctic Peninsula, and provides a common link for climate-related change on op- posite sides of the continent. If this trend persists, Lake Vanda should continue to rise and cool over the next 200 years until a new equilibrium lake level is achieved. Most likely, future lake rise will lead to isothermal con- ditions not conducive to thermohaline convection, resulting in a drastically different physical, biogeochemical, and biological structure than observed today. © 2016 Elsevier B.V. All rights reserved. Contents 1. Introduction.............................................................. 214 2. Lakesetting.............................................................. 215 2.1. Geography........................................................... 215 2.2. Geology............................................................. 215 3. Thelakelevelrecord.......................................................... 215 3.1. Last Glacial Maximum – GlacialLakeWright............................................ 215 3.2. Holocenelakelevels....................................................... 216 3.3. Recentlakelevelchange..................................................... 217 4. Changestophysicalcharacteristic.................................................... 217 4.1. Positionandtemperatureofconvectioncells............................................ 217 4.2. Formationoftheupperconvectioncell............................................... 217 4.3. Reductioninicethickness..................................................... 218 5. Changesinthelakewaterbalance.................................................... 219 ⁎ Corresponding author. E-mail addresses: [email protected] (D.N. Castendyk), [email protected] (M.K. Obryk), [email protected] (S.Z. Leidman), [email protected] (M. Gooseff), [email protected] (I. Hawes). http://dx.doi.org/10.1016/j.gloplacha.2016.06.007 0921-8181/© 2016 Elsevier B.V. All rights reserved. 214 D.N. Castendyk et al. / Global and Planetary Change 144 (2016) 213–227 5.1. OnyxRiverdischarge....................................................... 219 5.2. Interiorclimateandlakewaterlosses................................................ 220 5.3. Newbathymetryandwaterstoragecalculations........................................... 220 5.4. Newwaterbalancemodel..................................................... 221 6. ChangetotheLakeVandaecosystem................................................... 222 6.1. Planktoniccommunities...................................................... 222 6.2. Benthiccommunities....................................................... 222 7. Regionalclimatechangeoverthepast80years............................................... 223 8. PredictionforthefutureLakeVanda................................................... 224 9. Conclusions............................................................... 225 Acknowledgements............................................................. 225 References.................................................................. 225 1. Introduction Canada (Mueller et al., 2009; Veillette et al., 2010; Paquette et al., 2015), Signy Island, Antarctica (Quayle et al., 2002), and the Antarctic Peninsu- Lakes are widely regarded as sentinels of climate change la (Lyons et al., 2006). Perennially ice covered, meromictic lakes in the (Williamson et al., 2009; Adrian et al., 2009; Schindler, 2009)asmany interior of Antarctica also show evidence of changing climate through of their physical, chemical and biological properties are sensitive to var- changes in water balance leading to increases in lake level. Lake iations in meteorological and hydrological conditions. These include ice Untersee in the Schirmacher Oasis (71°S) showed little change from phenology (Magnuson et al., 2000; Duguay et al., 2006) and surface 1939 to 1981, and then increased in depth between 1981 and 1991 water temperature (Austin and Colman, 2007; Schneider et al., 2009; (Wand et al., 1997). The level of Lake Wilson in the Darwin Valley of Schneider and Hook, 2010). The water level and salinity of endorheic Southern Victoria Land (80°S) increased 25 m between 1975 and (i.e., closed-basin, with no outflow) lakes are particularly sensitive to 1993, a 54% increase in volume (Webster et al., 1996). Lake levels in changes in the lake water balance (Adrian et al., 2009; Schneider et al., the McMurdo Dry Valleys (77°S; MDV) of Southern Victoria Land, 2009). Lakes are the lowest points within watersheds, and as such, re- have risen substantially and steadily since the first observations were ceive and integrate climate effects across landscapes, while the wide made by Sir Robert Falcon Scott's Discovery Expedition in 1903 (Chinn, geographical distribution of lakes provides the ability to detect and 1993; Bomblies et al., 2001; Scott, 1905). Detailed records of level monitor climate change at regional, continental, hemispheric, and glob- change in the MDV lakes have been made for nearly 50 years, through al scales (Williamson et al., 2009). the efforts of the New Zealand Antarctic Program (1970-present), and Polar and sub-polar lakes in particular are considered some of the the United States, McMurdo Dry Valleys, Long Term Ecological Research most sensitive to climate change (Vincent et al., 1998; Lyons et al., (MCM-LTER) Program (1993-present), and provide one of longest and 2006; Adrian et al., 2009; Williamson et al., 2009; Vincent et al., 2008; richest environmental datasets on the continent. Paquette et al., 2015). With a large volume of glacial ice and snow stored Situated in the Wright Valley (Fig. 1), Lake Vanda is the deepest and in watersheds, and summer air temperatures near the melting point of largest of the MDV lakes, and has the most complete direct record of water, these lakes quickly respond to slight changes in the energy bal- level and inflow volume. This paper seeks to summarize recent changes ance. Researchers have found evidence of warming trends in lakes in in this lake, place these changes in the context of historic changes, link Alaska, USA (Engstrom et al., 2000; Surdu et al., 2014), Ellesmere Island, these changes to local and regional drivers of climate change, and Fig. 1. Location map showing the position of Lake Vanda between the Wright Upper Glacier (an outlet of East Antarctic Ice Sheet from the Polar Plateau) to the west and the Wright Lower Glacier (an outlet of the Wilson Piedmont Glacier) abutting the Ross Sea to the east. The Onyx River flows inland (westward) for 28 km, beginning at Lake Brownworth and ending at Lake Vanda (Shaw and Healy, 1980). Download English Version: https://daneshyari.com/en/article/4463281 Download Persian Version: https://daneshyari.com/article/4463281 Daneshyari.com.
Details
-
File Typepdf
-
Upload Time-
-
Content LanguagesEnglish
-
Upload UserAnonymous/Not logged-in
-
File Pages3 Page
-
File Size-