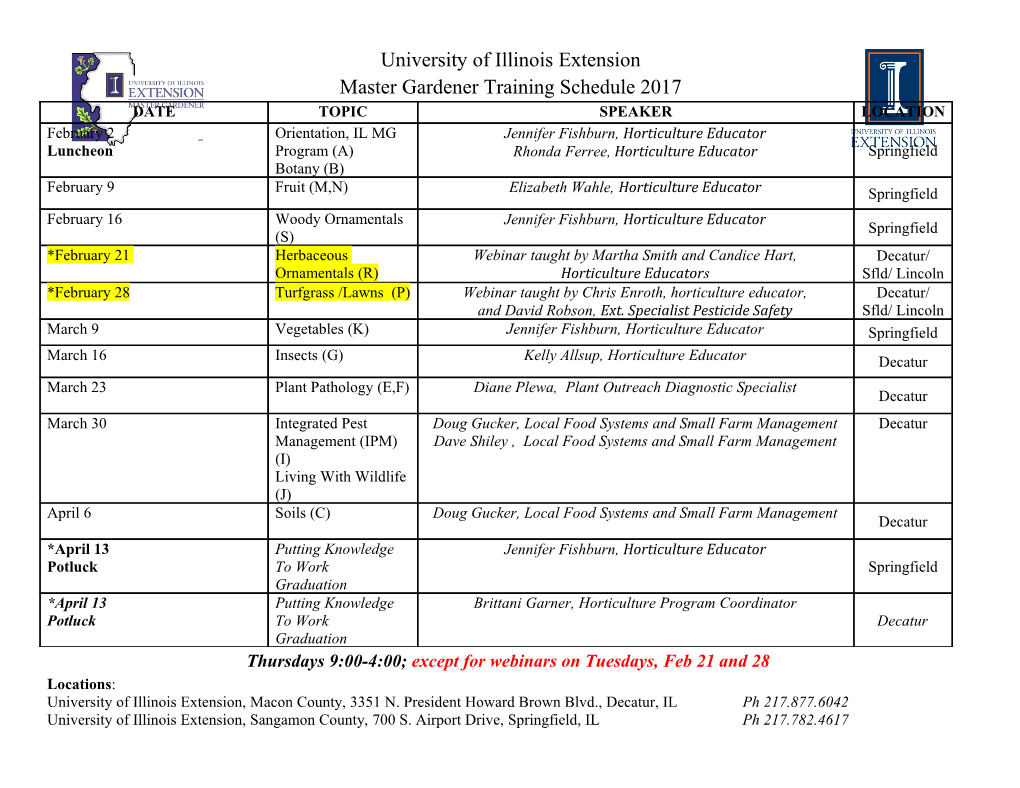
Total Synthesis and Chemoproteomics Connect Curcusone Diterpenes with Oncogenic Protein BRAT1 Chengsen Cui1†, Brendan G. Dwyer2†, Chang Liu1, Daniel Abegg2, Zhongjian Cai1, Dominic Hoch2, Xianglin Yin1, Nan Qiu2, Jieqing Liu3, Alexander Adibekian2*, Mingji Dai1* 1Department of Chemistry and Center for Cancer Research, Purdue University, West Lafayette, IN 47907, United States 2Department of Chemistry, The Scripps Research Institute, 130 Scripps Way, Jupiter, FL 33458, United States 3School of Medicine, Huaqiao University, Quanzhou 362021, P. R. China Correspondence and requests for materials should be addressed to M. D. (email: [email protected]) and A. A. ([email protected]) †Contributed equally. Abstract: Natural products are an indispensable source of lifesaving medicine, but natural product-based drug discovery often suffers from scarce natural supply and unknown mode of action. The study and development of anticancer curcusone diterpenes fall into such a dilemma. Meanwhile, many biologically- validated disease targets are considered “undruggable” due to the lack of enzymatic activity and/or predicted small molecule binding sites. The oncogenic BRCA1-associated ATM activator 1 (BRAT1) belongs to such an “undruggable” category. Here, we report our synthetic and chemoproteomics studies of the curcusone diterpenes that culminate in an efficient total synthesis and the identification of BRAT1 as a cellular target. We demonstrate for the first time that BRAT1 can be inhibited by a small molecule (curcusone D), resulting in impaired DNA damage response, reduced cancer cell migration, potentiated activity of the DNA damaging drug etoposide, and other phenotypes similar to BRAT1 knockdown. 1 Natural products have been valuable sources and inspirations of lifesaving drug molecules1. Their accumulated evolutionary wisdom together with their structural novelty and diversity makes them unparalleled for novel therapeutic development. However, their natural scarcity, structural complexity, and unknown mode of action often hamper their further development in the drug discovery pipeline. Total synthesis is frequently employed to solve the material supply and chemical probe synthesis for comprehensive biological profiling and target identification2. Meanwhile, many biologically validated disease targets are considered as “undruggable” from a chemical standpoint due to the lack of enzymatic activity and/or small molecule binding sites3. The BRCA1-associated ATM activator 1 (BRAT1) protein has been validated as an oncogenic protein involved in various cancers but belongs to the “undruggable” category with no known small molecule inhibitors to date. Herein, we report a collaborative effort in the total synthesis and chemoproteomics profiling of curcusone natural products which reveals BRAT1 as a key cellular target and validated curcusone D as the first BRAT1 inhibitor. The curcusone diterpenes (Fig. 1A) were isolated from Jatropha curcas, a widely used ingredient in traditional remedies for a variety of ailments including cancer. Structurally, they share a characteristic [6-7-5] tricyclic skeleton with the daphnane and tigliane diterpenes4. Curcusones A-D (1a-d), isolated by Clardy and co-workers in 1986, were unambiguously identified as two epimeric pairs at the C2 position5. Since then, around thirty curcusone molecules have been isolated including curcusones F-J6, which lack the dienone moiety in the seven-membered ring. Structurally rearranged analogs like spirocurcasone (3) and dimeric analogs such as dimericursone A (2a) and dimericursone B (2b) were discovered recently7,8. Among them, 1a-1d exhibited low micromolar IC50 values against a broad spectrum of human cancer cell lines6. However, their mode of action remained unknown and no total syntheses of 1a-1d were reported prior to this study. 2 A Curcusone Natural Products 12 18 13 11 16 O H 14 O H O H O H O H 15 H 2 9 8 H 2 H H H H 1 Me HO Me Me Me Me Me 7 17 Me Me Me OH 19 Me 3 5 6 Me Me HO Me MeO Me MeO OH O 20 O O O O Me Curcusone A (1a: 2S) Curcusone C (1c: 2R) Curcusone B (1b: 2R) Curcusone D (1d: 2S) Curcusone E (1e) Curcusone F (1f) Curcusone G (1g) anti cancer cell proliferation and antimetastatic Me Me Me O Me O H H O O H O H H H O O H H Me H Me Me H Me Me Me Me O H H H Me Me Me Me O O O O H Me Me Me Me Me H O O Me Me Curcusone I (1i: 2S) Curcusone H (1h) Curcusone J (1j: 2R) Spirocurcasone (3) putative structures Dimericursone A (2a) Dimericursone B (2b) B Our Previous Total Syntheses of Curcusones I and J MeO C Me 2 MeO2C O H TBSO Me H H TBSO TBSO TBSO H OTBS C H H OTBS H Me Au(I) Me O O O O Diels-Alder Me Me O Me Me TBSO 4 HO 5 TBSO putative (±)-1i & 1j Au-catalyzed furan formation/furan-allene [4+3] 6 7 (21 steps) C Synthetic Studies toward the Curcusones by Stoltz et al. TBSO O 6 steps TBSO OTBS OTBS HO Me H Me Br 5 steps H H 8 Me 10 O DIBAL-H HO Me Me + O OH 2 steps O Me HO Me H Me Suzuki coupling Cope Br BPin 13 14 OH cyclopropanation 12 rearrangement o 9 11 PhH, 50 C 31% from 12 D Retrosynthetic Analysis O H O H O H O H Pd, CO O H 14 H R2 Me H Me H Me H Me 10 2 7 or O 9 8 H Me R1 6 RCM or Me Carbonylative X Me Y OTf O O Aldol Condensation O O Coupling Curcusones A-D 15 (Y = H or halide) 16 17 18 (1a-d) Claisen rearrangement biomimetic Diels-Alder- cheletropic elimination O HO O 14 14 RO O 8 + Dimericursone A (2a) Me Me Me 8 X X Dimericursone B (2b) (S)-(-)-perillaldehyde 21 20a (R = H) O ($1.1/g, Sigma-Aldrich) X = masked aldehyde 20b (R = Et) 19 Fig. 1. Curcusone Diterpenes. (A) Selected curcusone diterpenes and their analogs. (B) Our total synthesis of the putative structures of curcusones I and J. (C) Synthetic efforts toward the curcusones from the Stotlz group. (D) Our retrosynthetic analysis of curcusones A-D. 3 While the closely related daphnane and tigliane diterpenes have attracted a significant amount of synthetic interest9-13, the curcusone molecules have surprisingly received little attention despite their therapeutic potential. In 2017, we reported the first total syntheses of the putative structures of 1i and 1j in 21 steps (Fig. 1B), ultimately leading to the conclusion that the originally proposed structures of both 1i and 1j were incorrect14. Our synthesis involves a gold-catalyzed tandem furan formation and furan-allene [4+3] cycloaddition to build the 5,7-fused ring system with an oxa bridge and a Diels-Alder reaction to construct the 6-membered ring. In 2019, Stoltz and co-workers reported their studies toward synthesizing 1a-1d (Fig. 1C)15-17. Their approach features a divinylcyclopropane-cycloheptadiene rearrangement to forge the 7-membered ring and reached advanced intermediate 14 after 12 steps from 8. Total Synthesis and Probe Synthesis. Our ongoing interest in natural products that can covalently modify cellular proteins18 prompted us to continue pursuing the total synthesis and target identification of 1a-1d with an electrophilic cycloheptadienone moiety. This unique structural feature could allow them to form a covalent bond with nucleophilic residues of certain cellular proteins19. Previous cytotoxicity studies found that reduction and/or oxidation of the C6-C7 double bond greatly reduced their anticancer activity6. As such, an approach allowing variation of the C6 and C7 substituents would be highly desirable. We envisioned 15 as an advanced intermediate (Fig. 1D). α-Halogenation followed by two methylation reactions would lead to 1a and 1b, which could be oxidized to 1c and 1d via a-hydroxylation. A ring closing metathesis (RCM) or an intramolecular aldol condensation was planned to form the 7-membered dienone (16/17®15). Both 16 and 17 could be prepared from vinyl triflate 18 via Pd-catalyzed carbonylative cross couplings. At this stage, disconnection of the C9-C10 bond could break 18 into two simple pieces, but a direct intermolecular C-C bond formation to construct such a bond is challenging. Thus, we opted for a Claisen rearrangement to forge this C-C bond in a stereoselective manner and designed 19 with a masked aldehyde as the Claisen rearrangement precursor. 19 could be assembled from simple building blocks 20a/20b and 21. The latter could be derived from a cheap chiral pool molecule (S)-(−)-perillaldehyde 8. 4 OHC O OH OHC 1. TBSOTf, NEt3 2. NaBH4 HO 3. O CH2Cl2, 0 °C to r.t. EtOH/CH2Cl2 20a then HC(OEt)3 Me 0 °C to r.t. Me PPh3, DEAD, THF Me Me BF3•OEt2, 0 °C EtO OEt 73% over 2 steps EtO OEt 0 °C to 50 °C O EtO OEt 8 22 23 24 (S)-(-)-perillaldehyde no purification OEt FeCl3 4. DMF O 27 6. FeCl (2-MeTHF) H H 5. H 3 OEt H 140-150 °C O Li EtO OH TMSCl Me Me H Me H H 48% Me OEt TMEDA OEt toluene, r.t. O+Et over 2 steps EtO OEt O THF, -78 °C O 39% over 2 steps O OH 25 26 28 29 (X-ray) no purification H H O O O H O H 8. Pd(dppf)Cl2 FeCl3 7. I2, DMAP Me4Sn, CuI H Me H Me H Me H O Me OFeCl2 pyr./CH2Cl2 DMF, 60 °C Me r.t. I 45% over 2 steps O O O O 17 30 15 31 no purification O H O H O H O H 9. KHMDS, MeI 10. KHMDS H Me THF, -78 °C 2 H Me MoOPH 2 H Me 11.
Details
-
File Typepdf
-
Upload Time-
-
Content LanguagesEnglish
-
Upload UserAnonymous/Not logged-in
-
File Pages18 Page
-
File Size-