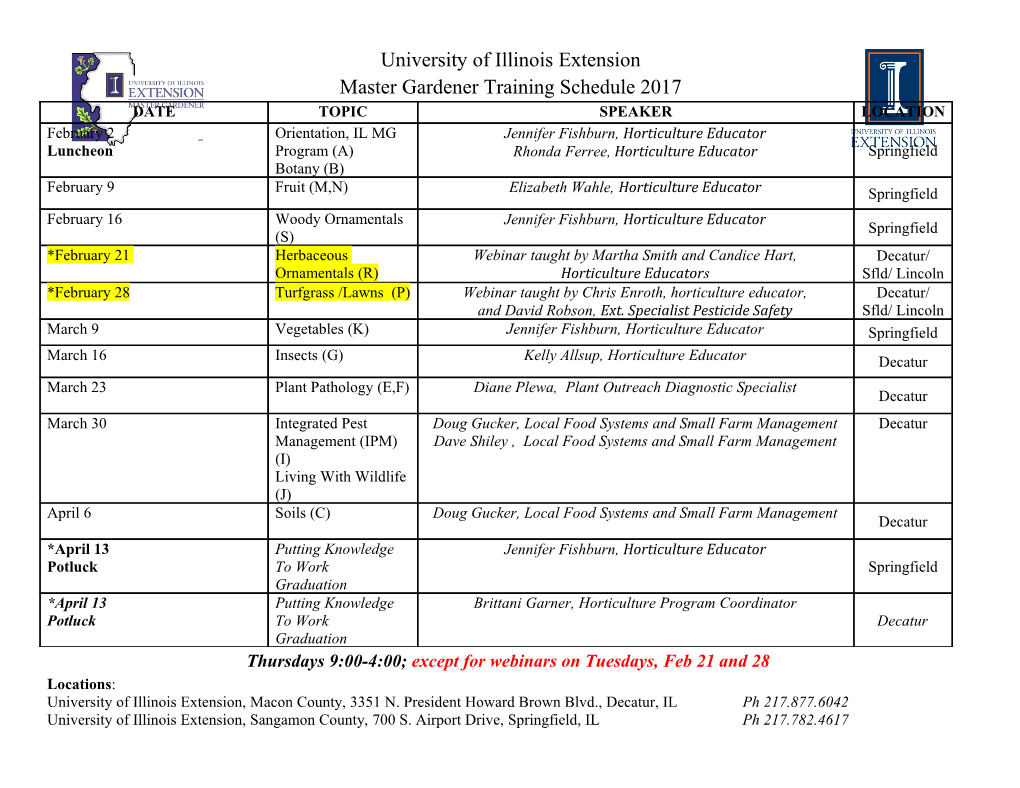
Hydrogen Isotope Transport and Separation via Layered and Two- Dimensional Materials DISSERTATION zur Erlangung des akademischen Grades Doktor der Naturwissenschaften (Dr. rer. nat.) am Bereich Mathematik und Naturwissenschaften der Technische Universität Dresden vorgelegt von M.Sc. Yun An geboren in Shanxi, China Erstgutachter: Prof. Dr. Thomas Heine Zweitgutachter: Prof. Dr. Ralf Tonner Tag der Verleihung: 19 April 2021 Dresden, 19 Januar 2021 Tag der mündlichen Prüfung: 19 April 2021 Die Dissertation wurde in der Zeit von Oktober 2016 bis Januar 2021 an der Technischen Universität Dresden unter der Betreuung von Prof. Dr. Heine durchgeführt. ii Abstract The enrichment of heavy hydrogen isotopes (deuterium, tritium) is required to fulfill their increasing application demands, e.g., in isotope related tracing, cancer therapy and nuclear reaction plants. However, their exceedingly low natural abundance and the close resemblance of physiochemical properties to protium render them extremely difficult to be separated. In this thesis, we investigate hydrogen isotope transport and separation via layered and two-dimensional materials. Three different theoretical challenges are undertaken in this work: (1) identification of the transported hydrogen species (proton H+ or protium H atom) inside interstitial space of layered materials (hexagonal boron nitride, molybdenum disulfide and graphite) and elucidation of their transport mechanism; (2) separation of hydron (proton H+, deuteron D+, and triton T+) isotopes through vacancy-free graphene and hexagonal boron nitride monolayers; (3) capture of the extremely rare light helium isotope (3He) with atomically thin two- dimensional materials. In the case of hydrogen transport, the essential challenges are investigation of its mechanism as well as the identification of transported particles. Regarding the case of hydron isotope separation, the essential questions are whether or not pristine graphene is permeable to the isotopes, and how quantum tunneling and topological Stone-Wales 55-77 defects affect their permeation and separation through graphene. In the last case, to capture the light helium isotope, quantum tunneling, which favors the lighter particles, is utilized to harvest 3He using graphdiyne monolayer. Our results provide novel theoretical insights into hydrogen particle transport inside the interstitial space of van der Waals materials; they uncover the mechanism of hydron isotope separation through 2D graphene and hexagonal boron nitride monolayers; and they predict the influence of pure quantum tunneling on the enrichment of 3He through graphdiyne membrane. iii iv Index of Abbreviations 1D one-dimensional 2D two-dimensional 3D three-dimensional 5MR 5-membered ring 6MR 6-membered ring 7MR 7-membered ring AFM atomic force microscope BO Born-Oppenheimer BOMD Born-Oppenheimer molecular dynamics BZ Brillouin zone CAQS chemical affinity quantum sieving CC coupled cluster CCSD(T) coupled cluster with single and double excitations, triple excitations estimated using perturbation theory CSVR canonical sampling through velocity rescaling CV collective variable CVD chemical vapor deposition DFT density functional theory D2O heavy water FES free energy surface GGA generalized gradient approximation GS Girdler-Sulfide GDY graphdiyne GY graphyne h-BN hexagonal boron nitride v HF Hartree-Fock KQS kinetic quantum sieving KS Kohn-Sham LDA local density approximation MetaD metadynamics MD molecular dynamics MoS2 molybdenum disulfide MP2 second order Møller–Plesset perturbation theory NQE nuclear quantum effect PBE Perdew–Burke-Ernzerhof PES potential energy surface PIMD path-integral molecular dynamics SE Schrödinger equation SW Stone-Wales TEM transmission electron microscopy vdW van der Waals WKB Wentzel-Kramers-Brillouin WT-MetaD well-tempered metadynamics ZPE zero-point energy vi List of Symbols D+ deuteron Dd self-diffusion coefficient E total energy Ea activation energy f(p) momentum probability distribution of particles H+ proton 3He light helium isotope 4He helium h Planck constant ℏ reduced Planck constant k wave number kB Boltzmann constant kf force constant kr rate constant T(E) transmission coefficient T temperature T+ triton Umax maximum energy barrier height u total particle flow uclass classical particle flow utunnel particle flow due to quantum tunneling β inverse of temperature, β=1/kBT vii viii Contents Abstract iii Index of Abbreviations v List of Symbols vii 1. Introduction 1 1.1 Hydrogen Isotope Separation ························································ 1 1.1.1 State-of-the-art Industrial Deuterium Enrichments ························ 2 1.1.2 Quantum Sieving ······························································· 3 1.1.3 Novel Hydrogen Isotope Separation Techniques ·························· 6 1.2 Motivation and Objectives ···························································· 8 1.3 Outline ·················································································· 10 2. Theoretical Background 13 2.1 Born-Oppenheimer Approximation ················································ 14 2.2 Density Functional Theory ·························································· 16 2.2.1 Kohn-Sham Equations ························································ 16 2.2.2 Exchange-Correlation Energy ··············································· 17 2.2.3 Dispersion Correction for DFT ·············································· 19 2.3 Nuclear Quantum Effects ···························································· 22 2.3.1 Nuclear Schrödinger Equation··············································· 22 2.3.2 Quantum Tunneling ··························································· 24 2.4 Free Energy Calculations ···························································· 30 2.4.1 Free Energy Perturbation Theory ··········································· 30 2.4.2 Collective Variables ··························································· 32 2.4.3 Metadynamics·································································· 33 2.5 Non-Arrhenius Behavior ····························································· 37 ix 3. The Fundamental Properties of Layered and 2D Materials 39 3.1 Layered Materials ····································································· 39 3.2 Two-dimensional Materials ·························································· 40 3.2.1 Graphene ········································································ 40 3.2.2 Hexagonal boron nitride ······················································ 44 3.2.3 Molybdenum Disulfide ······················································· 45 Part Ⅰ 47 Hydrogen Particle Transport in the Interstitial Space of Layered Materials 47 4. Hydrogen Transport in Interstitial Space of Layered h-BN, MoS2 and Graphite 49 4.1 Introduction ············································································ 49 4.2 Methodology ··········································································· 51 4.3 Calculation Details ···································································· 52 4.4 Results ·················································································· 54 4.4.1 Structural properties of proton and protium in h-BN, MoS2, and graphite ·················································································· 56 4.4.2. Diffusion process of proton and protium inside h-BN, MoS2, and graphite ········································································ 59 4.4.3 Can H atoms Recombine to H2 Molecules in the Interstitial Space of Layered Materials? ··························································· 67 4.5 Conclusions ············································································ 69 Part Ⅱ 71 Quantum Sieving Isotopes via Two-dimensional Materials 71 5. Hydron Isotope Separation Through Graphene and h-BN Monolayers 73 5.1 Introduction ············································································ 74 5.2 Methodology ··········································································· 77 5.3 Calculation Details ···································································· 78 5.4 Results ·················································································· 80 5.4.1 Hydron Isotope Permeate Through Graphene and h-BN Monolayers · 80 x 5.4.2 Influence of Hydrogenation ·················································· 83 5.4.3 Discussions ····································································· 85 5.5 Revisit Arrhenius Equation ·························································· 87 5.6 Conclusions ············································································ 92 6. Graphdiyne Monolayer as Efficient Quantum Sieve for Helium Isotopes 95 6.1 Introduction ············································································ 95 6.2 Models and Methods ································································· 97 6.3 Results ·················································································· 99 6.4 Conclusions ·········································································· 102 7. Concluding Remarks 105 7.1 Summary ············································································· 105 7.2 Further Work ········································································· 107 Appendix 109 List of Publications 115 Conference Contributions 117 Acknowledgements 119 Bibliography 121
Details
-
File Typepdf
-
Upload Time-
-
Content LanguagesEnglish
-
Upload UserAnonymous/Not logged-in
-
File Pages152 Page
-
File Size-