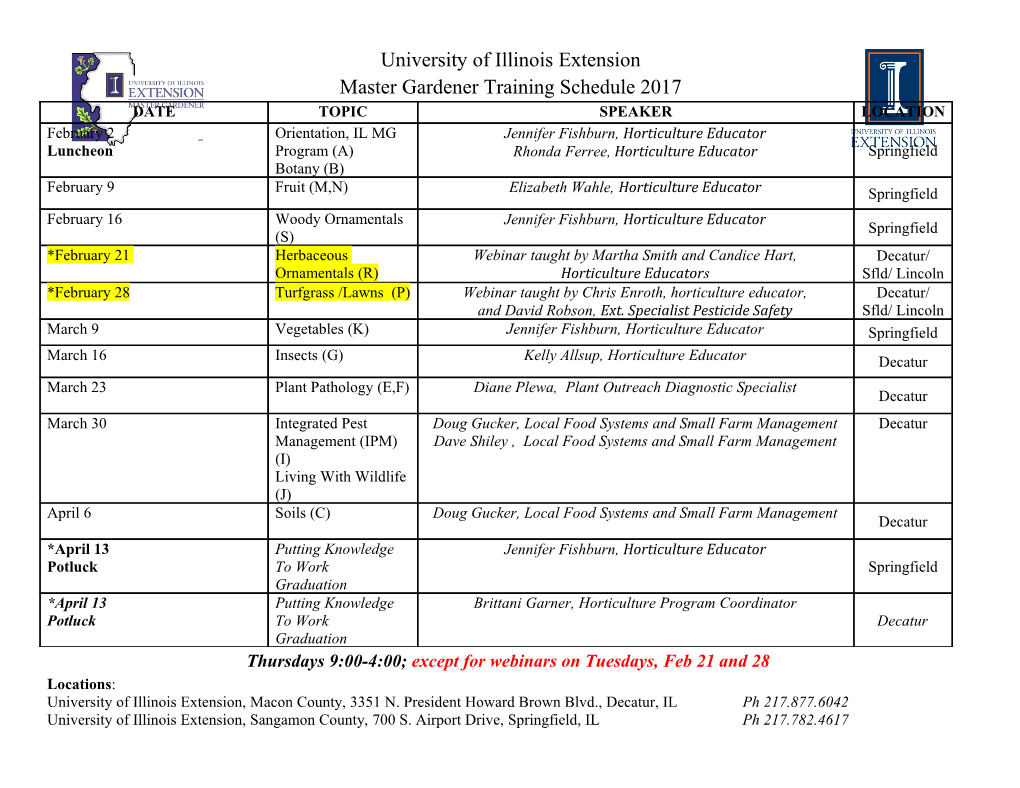
1 THE ORIGIN OF ATOMIC EMISSION LINES AND THE STRUCTURE OF THE ATOM Stephen D Pastor Department of Chemistry Pace University Pleasantville, NY 10570 [email protected] Although Newton discovered in 1666 that the various wavelengths constituting white light refracted at different angles when passed through a prism to give a colored spectrum, the use of spectroscopy to identify elements and molecules in the solar system and beyond developed late in the 19th century.1 Lockyer, for example, in his textbook Elements of Astronomy published in 1871 devoted several pages to a discussion of the analysis of spectra of the sun, planets, stars, and nebulae.2 The somewhat early independent development of the sciences of physics and chemistry to that of atomic and molecular spectroscopy has led to the sometimes confusing conventions used for the designation of the ionization state of an atom and its corresponding emission line. The OIII filter used to photograph particular emission lines of planetary and other emission nebulae or visually enhance their telescopic detail provides a good example of differing conventions used for ionization (or oxidation) states of an atom and its corresponding emission lines. In the convention adopted in the discipline of atomic spectroscopy,3 the unionized or neutral (unoxidized) state of an atom is designated by the symbol of that element followed by the Roman numeral I. For a neutral unionized oxygen atom the corresponding symbol is OI. The unionized state of an oxygen atom, OI, has its full complement of 8 electrons (orbital designations for the occupancy of the six electrons, 2 the electron configuration, would be 1s22s22p4 where the numerical prefix is the principle quantum number of the orbital; the lower case letter the type of orbital; and the superscript is the number of electrons in that orbital, vide infra)4. The unionized state or ground state of a hydrogen atom would be HI (electron configuration 1s1). Following this convention, mono-ionized oxygen would be designated OII and mono-ionized hydrogen is HII. The mono-ionized oxygen has only 7 electrons (1s22s22p3 electron configuration) whereas the hydrogen atom has no electrons (only the nucleus remains, which is simply a proton). Emission nebula are typically clouds of predominantly ionized hydrogen atoms and are often called HII regions. HII regions can often be seen visually with large amateur telescopes in galaxies such as Messier 33 in triangulum. Doubly ionized oxygen is designated by the spectroscopist as OIII and has only 6 electrons (1s22s22p2 electron configuration). Due to the fact that both doubly-ionized oxygen, OIII, and singly-ionized nitrogen, NII, have the same number of electrons (6 electrons in 1s22s22p2 orbitals), OIII and NII are isolectronic (they both have the same number of electrons and electron configuration) and have similar fine structural levels in their emission spectra, although the emission lines are expected to be of different energy (nuclear charge is different and the resultant electron orbital energy levels will be different).5 Standard notation in the chemical and physical sciences denote the neutral or unionized state of an atom by the symbol of the element followed by a 0 in parentheses. The neutral state of oxygen and hydrogen would be denoted as O(0) and H(0), respectively. The first ionized state of hydrogen and oxygen following this convention would be denoted H(I) and O(I), respectively. The doubly-ionized state of oxygen would be denoted O(II). There are several other commonly accepted conventions found in the chemical and physical literature for ionized states. Doubly-ionized (oxidized) oxygen will commonly be found denoted in the literature as O(II), O2+, O++ or OII. Returning now to our OIII filter, the symbol OIII represents doubly-ionized oxygen since the context of the description is that of a spectral emission line filter, that is, the language and discipline of spectroscopy. If reference was being made to the chemistry or physics of doubly-ionized oxygen is would be correct to use the symbol O(II). Due to the fact that OIII and O(II) both designate doubly-ionized oxygen, one may find both conventions on the same page of a textbook dependent upon the context of the discussion. We will see later that a further convention is used to designate whether a particular emission is “allowed or forbidden,” vide infra. Before we discuss the origin of atomic emission lines, we should answer the question “why can hydrogen and other gaseous elements exist in nebulae and interstellar space as free atoms rather than molecules as on earth?” The reaction of two hydrogen atoms, H. (H denoting hydrogen and the dot denoting the unpaired electron; 1s1 orbital occupancy), would be expected to form molecular hydrogen, H2 or H-H (where the line denotes a bond between the hydrogens composed of two shared electrons of opposite spin, vida infra). H + H → H2 3 However, for this reaction to proceed irreversibly requires a third body to carry off the excess energy released upon bond formation. If two hydrogen atoms collide and a third body is not present to carry off this excess energy, the two hydrogen atoms merely fly apart.6 On earth the density of our atmosphere is high and a three-body collision is statistically possible. Thus hydrogen atoms quickly combine to form molecular hydrogen with the third body carrying off the excess energy. In contrast to the earth, the densities of hydrogen atoms in interstellar regions can be a low as 103 m-3. At low densities, the collision of one hydrogen atom with another may occur on average at intervals of 1013 seconds, almost a million years!7 The possibility of a three body collision would be extremely low. Indeed, the formation of molecular hydrogen that is present in interstellar space is suggested to be formed on the surface of interstellar dust grains.8 In 1859 Kirchoff put forth a series of postulates now known as Kirchhoff’s laws that states 1) bright spectral lines (emission lines) are produced in a hot diffuse gas, 2) adsorption lines are produced when a source of continuous light is placed in front of a cool diffuse gas, and 3) a continuous spectra is produced by a hot dense gas or solid.9 To the first approximation, atomic spectra involve emissions (or corresponding absorptions) due to electronic transitions between orbitals of an atom (an electron jumping or falling down between two orbitals.9 As we are interested in the origin of emission lines, we will focus on the energy given off as light of a particular wavelength when an electron falls from a higher to lower energy orbital of an atom. Molecular spectra involve transitions between various vibrational and rotational levels of a molecule and are outside the scope of our discussion.10 The hydrogen alpha filter is popular among astrophotographers. The hydrogen alpha emission is denoted by the symbol for the element hydrogen followed by a Greek alpha, Hα, which often is also denoted by a subscript, Hα. The origin of emission lines is the falling of an electron from a higher energy orbital to a lower energy orbital with the excess energy being given off as light or energy of a particular wavelength. The energy of a particular orbital of hydrogen is quantized, that is, the orbital has a discrete energy value that is determined solely by the quantum numbers of a particular orbital. Therefore the difference in energy between two orbitals has a discrete value.11 It follows that the energy given off when an electron falls from a higher energy orbital to a lower energy must have a discrete value. The relationship between the value of the energy given off and it’s corresponding frequency is E = hν, where E is the energy, h is Planck’s constant, and ν is the frequency (sec-1). The corresponding wavelength of the emission is λ = c/ν, where λ is the wavelength, ν is the frequency, and c is the speed of light.12 Substituting the wavelength for frequency, we obtain E = hc/λ that shows there is an inverse relationship between energy and wavelength, in other words, the shorter the wavelength is of the emitted light the higher it is in energy. In common usage, the wavelength λ is usually expressed in nanometers, nm , or the Angstrom unit, Å, and less often the picometer, pm. A nanometer is equal to 1 x 10-9 meters or 1 x 10-7 centimeters, an Angstrom is equal to 1 x 10-10 meters or 1 x 10-8 centimeters, and a picometer is equal to -12 -10 1 x 10 meters or 1 x 10 centimeters; therefore 1 nm =10 Å = 1000 pm. Thus the Hα emission line is at 656.2808 nm, 6562.808 Å, or 656280.8 pm.13 To understand the spectroscopy of the hydrogen atom, we need to have a basic understanding of the general structure of an atom. We will start with the Bohr model of 4 the atom which was developed at the turn of the 20th century. The Bohr model of the atom consists of a positively charged nucleus with negatively charged electrons orbiting around it. The nucleus of an atom is composed of positively charged protons and uncharged neutrons. In a neutral unionized atom the total number of negatively charged electrons equals the number of positively charged protons leading to a net charge of zero. The atomic number of an element refers to the number of protons in the nucleus. The atomic weight of an element or atom refers to the number of protons and neutrons in the nucleus.
Details
-
File Typepdf
-
Upload Time-
-
Content LanguagesEnglish
-
Upload UserAnonymous/Not logged-in
-
File Pages17 Page
-
File Size-