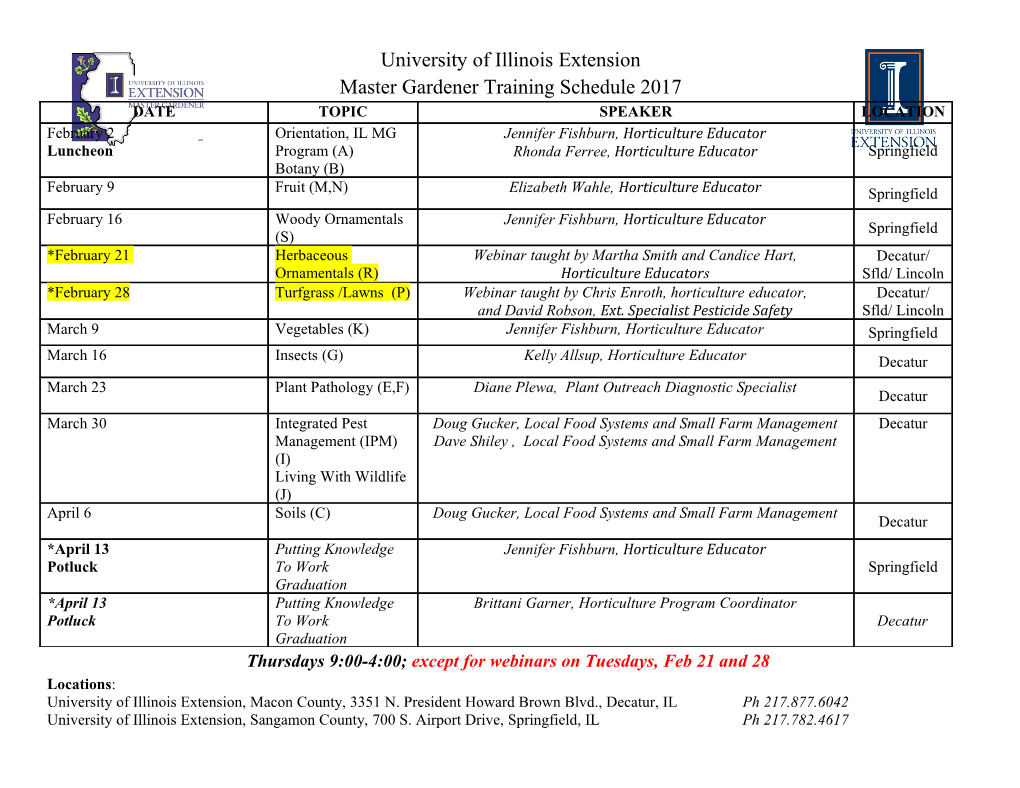
HYBRID SUPERCONDUCTING QUANTUM COMPUTING ARCHITECTURES by Matthew A. Beck A dissertation submitted in partial fulfillment of the requirements for the degree of Doctor of Philosophy (Physics) at the UNIVERSITY OF WISCONSIN–MADISON 2018 Date of final oral examination: 6/21/18 The dissertation is approved by the following members of the Final Oral Committee: Robert F. McDermott, Professor, Physics Mark Saffman, Professor, Physics Mark A. Eriksson, Professor, Physics Maxim G. Vavilov, Professor, Physics Mikhail Kats, Assistant Professor, Electrical and Computer Engineering ProQuest Number:10838041 All rights reserved INFORMATION TO ALL USERS The quality of this reproduction is dependent upon the quality of the copy submitted. In the unlikely event that the author did not send a complete manuscript and there are missing pages, these will be noted. Also, if material had to be removed, a note will indicate the deletion. ProQuest 10838041 Published by ProQuest LLC ( 2018). Copyright of the Dissertation is held by the Author. All rights reserved. This work is protected against unauthorized copying under Title 17, United States Code Microform Edition © ProQuest LLC. ProQuest LLC. 789 East Eisenhower Parkway P.O. Box 1346 Ann Arbor, MI 48106 - 1346 © Copyright by Matthew A. Beck 2018 All Rights Reserved i Abstract Quantum computing holds the promise to address and solve computational problems that are otherwise intractable on a classical, transistor based machine. While much progress has been made in the last decade towards the realization of a scalable superconducting quantum processor, many questions remain unanswered. The work contained in this thesis addresses two equally important concerns; These are specifically that of quantum information storage and transfer and the scaling of current qubit control and readout methods. Superconducting quantum processors are exactly what their name implies: processors. While the goal is to eventually build a universal quantum computer, it is not unreasonable to envision near term quantum processors hard wired to perform specific computational tasks. This idea of compartmentalized quantum processing necessitates that the quantum results of a computation either be stored and/or transferred for latter / further use. A natural candidate to realize such a quantum memory is the neutral Rydberg atom. The hyperfine states of cesium atoms exhibit coherence times greater than 1 second while adjacent Rydberg energy levels have electric dipole transitions in the gigahertz regime; These properties make it a suitable candidate to realize a quantum memory and information bus between adjacent superconducting processors yielding an unprecedented ratio of coherence time to gate time. To realize such a computing architecture, the coherent coupling between a single Rydberg atom and superconducting bus resonator must first be demonstrated. This first half of this thesis details the development of a superconducting interface meant to realize strong coupling to a single Rydberg atom. To date, the experimental liquid Helium 4 K UHV cryostat has been built, characterized, ii and installed. Superconducting niobium coplanar waveguide (CPW) resonators have been designed and fabricated to facilitate strong coupling to the Rydberg atom through on-chip microwave field engineering. Additionally, the CPW resonators have been tailored to achieve quality factors above 104 at 4 K. The project is currently still on-going with single-atom trapping and state characterization near the 4 K chip surface under investigation. The second portion of this thesis details the development of a superconducting single flux quantum (SFQ) pulse generator for transmon qubit control. As the size of superconducting quantum processors scales beyond the level of a few tens of qubits, the control hardware overhead becomes untenable. For current technology based on microwave control pulses generated at room temperature followed by amplification and heterodyne detection, the heat load and physical footprint of the required classical hardware preclude brute force scaling to qubit arrays more than ∼ 100. The work contained herein details the development, fabrication, characterization and finally integration of a dc/SFQ driver with a transmon qubit on a single chip as a first step towards an all superconducting digital control scheme of quantum processors. Details of the multi-additive layer processing and fabrication required to realize these devices are discussed in the context of maintaining high (> 10 us) qubit coherent times and small superconducting resonator loss. To date, coherent qubit rotations have been achieved via application of SFQ pulses with pulse to pulse spacing aligned with subharmonics of the qubit frequency. Interleaved randomized benchmarking (RB) of SFQ driven single qubit gates realized are currently at 90% level. Future plans regarding a flip chip / multi-chip module approach to increasing gate fidelities will also be discussed. iii Acknowledgments I would like to take just a few lines to give thanks to some notable people without whom this thesis and the work contained herein would not be possible. First and foremost, I would like to extend my deepest gratitude to my advisor Dr. Robert F. McDermott. It has been with Robert’s guidance that I have learned how to be a better scientist. To my graduate student forefathers in the group, Dr. David Hover, Dr. Steven Sendelbach, Dr. Yuanfeng Gao and Dr. Guilhem Ribeill, I would like to take a sentence or two and extend many thanks to you all for your patience and guidance during my first few years in the group. The debt I owe you I hopefully can repay through forwarding these lessons to younger students. With regards to the hybrid atom–CPW project, I would like to thank the tireless efforts of Jonathan Pritchard, Joshua Isaacs and P.I. Mark Saffman of the atomic team. You three have been a pleasure to work with. This acknowledgment section (nor this thesis) could not have been completed without a mention of the Edward M. Leonard, Jr. The past few years working side by side in the lab and clean room have been the most enjoyable professional experience of my life. Your tireless efforts in always striving for cleaner samples, cleaner measurements and cleaner code have been an inspiration. Your leadership in the lab has be a shining example to all in just how much one person can achieve. I, as does the entire lab, owe you a great deal of gratitude. I also would like to take a moment and thank the cowboys of the McDermott Family Research Ranch, Alex, J.P., Nathan, Naveen, Ted, Brad, Ryan, and Joey. Thank you all for iv the many riveting discussions and helping to maintain a scalable lab. Raising a child through undergraduate and graduate study could not have been possible without the endearing love and support of my parents. I wish to give many thanks to my mother who has been a bedrock of patience and understanding and to my father who showed me what it means (and takes) to be a good man and father. And finally, Alyssa and Zoe. Alyssa, you have been the love of my life since we were kids. Thank you for everything you do everyday and showing me what happiness truly is. Zoe, watching you grow up into the wonderful young adult you are has been a privilege. I can only hope to teach you the number of lessons you have taught me. With that, this is for you, kid. v To my family. vi Contents Contents ....................................... vi List of Tables .................................... ix List of Figures ................................... x 1 Introduction ................................... 1 1.1PhysicalInformation............................ 2 1.2QuantumBits................................ 4 1.2.1 Qubit Metrics ............................ 5 1.3 Physical Qubit Realizations ........................ 7 1.3.1 NeutralAtoms........................... 8 1.3.2 Superconducting Qubits ...................... 9 1.3.3 Current Hybrid Quantum Computing Approaches ........ 12 2 Josephson Physics and Superconducting Circuit Fundamentals ... 14 2.1BasicPhenomena.............................. 15 2.1.1 RCSJModel............................. 17 2.1.2 Superconducting Quantum Interference Devices ......... 20 2.2QuantizationOfElectricalCircuits.................... 22 2.3 Superconducting Qubit Zoo ........................ 26 2.3.1 Phase qubit ............................. 26 2.3.2 Flux Qubit ............................. 27 2.3.3 Transmon.............................. 27 3 Circuit Quantum Electrodynamics ..................... 29 3.1 Cavity–Qubit Hamiltonian ........................ 30 3.1.1 Resonant Jaynes–Cummings Hamiltonian ............. 33 3.1.2 Dispersive Jaynes-Cummings Hamiltonian ............ 34 vii 3.2 Qubit-Cavity Bringup ........................... 37 3.2.1 Coupling The Qubit–Cavity System to the Outside World . 37 3.2.2 Qubit Signs of Life ......................... 41 3.2.3 Finding the Qubit Frequency ................... 45 3.2.4 Orthogonal Qubit Axis Control .................. 45 3.3 Qubit and Qubit Gate Benchmarking ................... 49 3.3.1 Characterizing and Calibrating the Qubit States ......... 51 3.3.2 Randomized Benchmarking of Qubit Gates ............ 53 4 Hybrid Atom–CPW cQED Experiment ................. 57 4.1ByTheNumbers.............................. 57 4.2 Experimental Apparatus .......................... 61 4.2.1 CryostatPerformance....................... 67 4.3 Superconducting CPW Resonator Design ................. 68 4.3.1 EngineeringtheQualityFactor.................. 71 4.3.2 MeasuringtheQualityFactor..................
Details
-
File Typepdf
-
Upload Time-
-
Content LanguagesEnglish
-
Upload UserAnonymous/Not logged-in
-
File Pages180 Page
-
File Size-