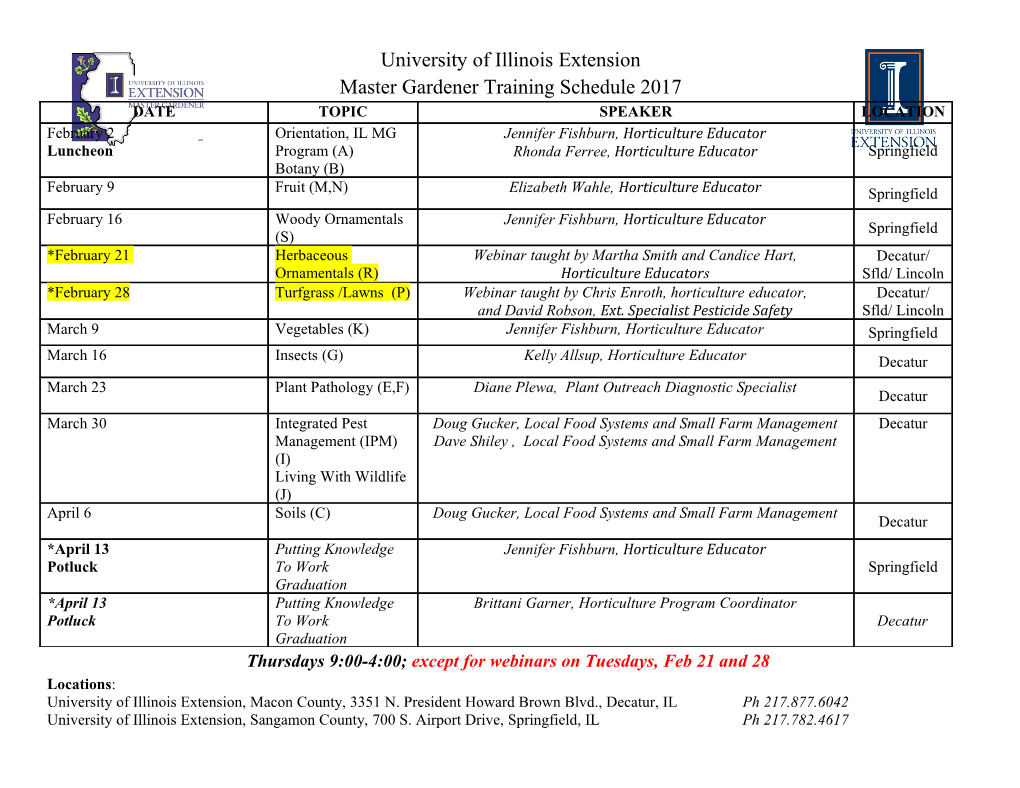
, Closing in on the origin of Galactic cosmic rays using multimessenger information Julia Becker Tjusa, Lukas Mertena,1 aRAPP Center and Theoretische Physik IV, Fakult¨atf¨urPhysik & Astronomie, Ruhr-Universit¨atBochum, 44780 Bochum, Germany bInstitute for Astro- and Particle Physics, University of Innsbruck, 6020 Innsbruck, Austria Abstract In cosmic ray physics extensive progress has been made in recent years, both concerning theory and obser- vation. The vast details in direct, indirect and secondary detections on the one hand provide the basis for a detailed modeling of the signatures via cosmic-ray transport and interaction, paving the way for the iden- tification of Galactic cosmic-ray sources. On the other hand, the large number of constraints from different channels of cosmic-ray observables challenges these models frequently. In this review, we will summarize the state-of-the art of the detection of cosmic rays and their secondaries, followed by a discussion what we can learn from coupling our knowledge of the cosmic-ray observables to the theory of cosmic-ray transport in the Galactic magnetic field. Finally, information from neutral secondaries will be added to draw a multimessenger-picture of the non-thermal sky, in which the hypothesis of supernova remnants as the dominant sources survives best. While this has been known since the 1930s, evidence for this scenario is steadily growing, with the first possible detection of hadronic signatures at GeV energies detected for three SNRs with Fermi. The existence of SNRs as PeVatrons, however, is not validated yet. The discussion of this and other open questions concerning the level of anisotropy, composition and spectral shape of the cosmic-ray energy spectrum is reviewed. Future perspectives of how to find the smoking cosmic-ray source gun concludes this review. Keywords: cosmic rays, astrophysical neutrinos, high-energy astrophysics, Milky Way, Supernova Remnants PACS: 98.35.-a, 98.35.Nq, 98.38.Dq, 98.38.Mz, 95.85.Ry, 95.85.Pw Contents 1 Introduction 4 2 Theory on signatures from cosmic-ray secondaries 5 2.1 Continuum signatures . .6 2.1.1 Hadronic secondaries . .6 arXiv:2002.00964v2 [astro-ph.HE] 7 May 2020 2.1.2 Leptonic secondaries . .8 2.1.3 Inverse Compton Radiation . 10 2.2 Line signatures from cosmic-ray impact . 12 2.3 The global picture of radiation signatures . 14 3 Cosmic rays and their secondaries: observations 17 3.1 Cosmic ray energy spectrum . 17 3.1.1 The cosmic-ray hip | the change in slope at R = 300 GV . 18 3.1.2 The knee and the iron knee . 20 Preprint submitted to Physics Reports May 11, 2020 3.1.3 The second cosmic-ray knee . 24 3.1.4 The cosmic-ray ankle . 25 3.1.5 The high-energy cut-off . 25 3.1.6 Summary . 26 3.2 Cosmic-ray composition . 26 3.2.1 Primary-to-secondary ratio . 26 3.3 (An)isotropy . 29 3.3.1 Dipole anisotropy . 29 3.3.2 Multipole anisotropy . 30 3.4 Leptons and antimatter . 35 3.5 Hadronic interaction signatures . 36 3.5.1 Photons . 37 3.5.2 Neutrinos . 40 4 Cosmic ray fundamental properties 44 4.1 Background theory . 45 4.2 Cosmic-ray acceleration . 49 4.2.1 Relation of electron and hadron spectra . 51 4.2.2 Stochastic acceleration . 51 4.3 Maximum energy . 56 4.3.1 Maximum energy from acceleration process . 57 4.3.2 Loss-dominated maximum energy . 58 4.3.3 Maximum energy for Galactic sources . 58 4.4 Diffusive transport . 61 4.4.1 Description of the diffusion coefficient within the Kolmogorov scale analysis . 61 4.4.2 Using spallation to determine the Galactic scalar diffusion coefficient . 72 4.5 Spectral evolution of the cosmic ray composition . 72 4.5.1 Cosmic-ray abundance . 75 4.5.2 MeV - TeV cosmic rays . 78 4.5.3 TeV - EeV range . 80 4.5.4 EeV - ZeV range . 80 4.6 Luminosity criterion . 80 4.6.1 Galactic sources . 82 4.6.2 Extragalactic sources . 84 4.7 (An)isotropy . 86 4.7.1 The dipole anisotropy . 86 4.7.2 The small-scale anisotropy . 89 4.8 Summary and Conclusions . 92 5 Multimessenger modeling 94 5.1 Numerical concepts of multimessenger modeling . 94 5.1.1 Numerical transport modeling . 95 5.2 Supernova remnants . 98 5.3 The Galactic sink: cosmic-ray propagation around the Sun . 105 5.4 Diffuse gamma-ray emission in the Milky Way . 109 5.4.1 Gas densities . 109 5.4.2 Spectral behavior and source distribution . 109 5.4.3 Magnetic field modeling . 111 5.4.4 Deviation from diffusive behavior . 115 5.4.5 Neutrino flux predictions . 116 5.4.6 Signatures from the Galactic wind . 116 2 6 Summary, Conclusions and Outlook 121 3 1. Introduction Source Region (acceleration/interaction) pions neutrinos/gammas H2+ chemical network vibration/rotation lines γ γ high-energy low-energy ν protons and other Photon, Neutrino and Hadron charged particles Detection Region interstellar γ gas Photons Neutrinos ν γ-ray telescope Protons etc. radio Earth telescope Myons neutrino and muon detector particle Pions ν accelerator ~ detector Kaons B airshower µ Molecules Milky Way Electrons/ particle shower Positrons (diffusion/advection/interaction) atmosphere Figure 1: Schematic picture of the non-thermal multimessenger emission from hadronic cosmic-ray sources in the Galaxy. Figure inspired by Wagner [460]. In 1934, 22 years after the first detection of cosmic rays, Baade and Zwicky [104] suggested supernova remnants as the primary sources of this high-energy radiation. With increasing knowledge on the cosmic-ray energy spectrum over the years, this hypothesis was strengthened, in particular as the class of supernova remnants in the Milky Way appears to be the only reasonable candidate to be able to explain the high flux of cosmic rays: it can deliver an intensity of around 1 particle per second and square-meter on Earth at 50 GeV particle energies, which can only be obtained when 10% of the energy released in supernova explosions∼ of massive stars in the Galaxy goes into cosmic-ray production. Still, more than 100 years after the first detection of cosmic rays, the mystery of their origin is subject to state-of-the-art research: how can supernova remnants reach energies up to or above 1015 eV? How can the observed large- and small-scale anisotropy level of 0:1% 0:01% be reproduced in the TeV { PeV range? Enormous progress has− been made in encompassing details of cosmic rays and their tracers during the past 10 15 years by putting a large variety of top observatories in operation. As for direct cosmic- ray measurements,− composition information about the cosmic-ray spectrum is known from GeV to >PeV energies, several breaks in addition to the long-known knee and ankle features have been detected and the observation of the anisotropy level in the TeV { PeV range has been established on small and large scales. Concerning the direct view on the sources, more than 80 Galactic TeV emitters have been identified and a diffuse high-energy neutrino flux was confirmed for the first time in 2013 [6], although not dominantly arising in the Galaxy. These many details of multimessenger information as summarized in Fig. 1 are both a blessing and a curse: as more and more details are revealed, the simplified models that have been used for many years in order to make the case for supernova remnants as primary source candidates for the cosmic-ray flux are 4 not able to reproduce all these details. At the same time, these insights have triggered the development of complex multimessenger methods and models. In this review article, the current state of the art of our knowledge and ignorance of Galactic cosmic-ray sources will be presented. In particular, primary cosmic-ray observables from MeV to EeV will be presented as well as information from secondary cosmic rays, produced in cosmic-ray interactions at the production site or in the interstellar medium on their way to Earth. This includes a (potentially) direct view of the sources via e.g. ionization signatures, neutrinos and gamma-rays as well as those pieces of information provided by cosmic rays themselves, i.e. spectrum, chemical composition and arrival directions. Here, astrophysical parameters of the source candidates and of the interstellar medium through which the propagation of cosmic rays needs to be modeled will be presented, in particular concerning the description of magnetic field configurations, gas densities, etc. The modeling of hadronic cosmic-ray interactions with cross-sections that match recent measurements at LHC is another aspect that needs to be considered in order to properly include hadronic secondary production. Finally, radiation from cosmic-ray electrons, in particular synchrotron radiation, Inverse Compton (IC) and bremsstrahlung (brems), on the one hand are used to investigate the acceleration environment. The high-energy signatures also need to be disentangled from hadronically produced gamma-rays. It is the aim of this article to show how recent advances in experimental setups for the detection of cosmic-ray signatures in combination with theoretical modeling start to shed light on the answer to one of the central questions in physics and astrophysics: what is the origin of cosmic rays? This review is organized as follows: a short review on the theoretical modeling of cosmic-ray electron and hadron interaction and radiation signatures in the context of non-thermal astrophysics is given in Section 2. Section 3 presents the state-of-the-art of cosmic-ray data, including the cosmic ray all-particle spectrum from GeV to EeV energies together with what is known about composition, (an-)isostropy and the detection of cosmic-ray interaction products, gamma-rays and neutrinos.
Details
-
File Typepdf
-
Upload Time-
-
Content LanguagesEnglish
-
Upload UserAnonymous/Not logged-in
-
File Pages136 Page
-
File Size-