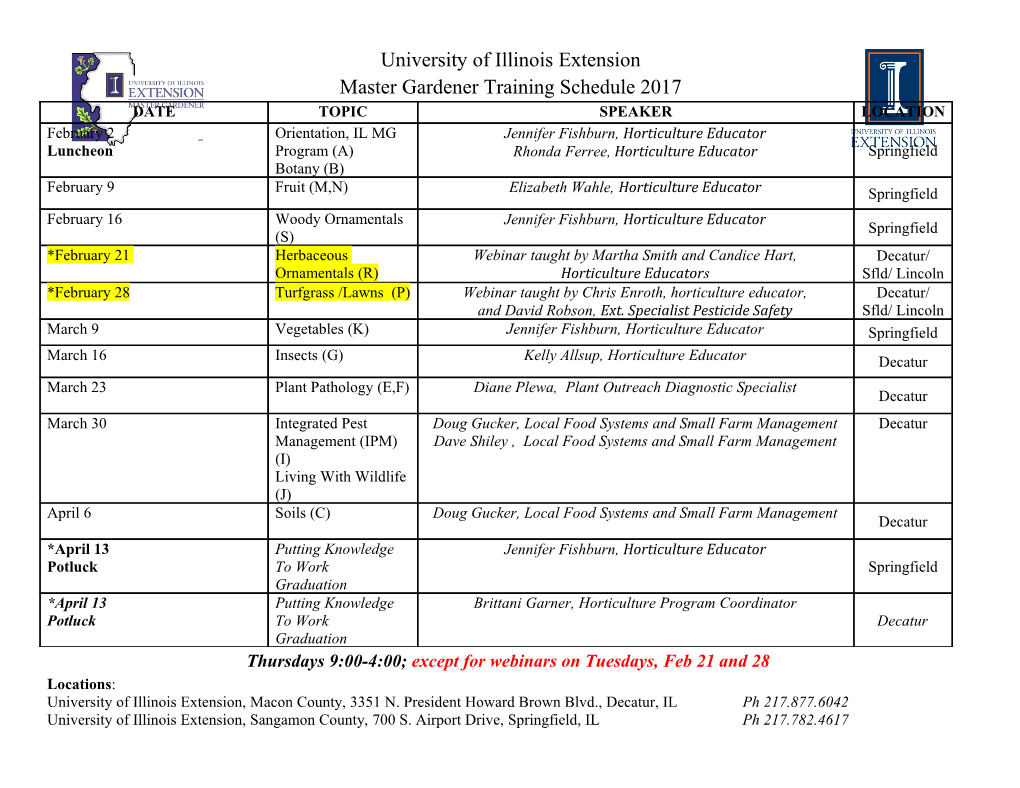
www.nature.com/scientificreports OPEN Animal multicellularity and polarity without Wnt signaling Quentin Schenkelaars1,2, Marine Pratlong 1,3, Laurent Kodjabachian 4, Laura Fierro- Constain1, Jean Vacelet1, André Le Bivic4, Emmanuelle Renard1 & Carole Borchiellini1 Received: 1 September 2017 Acquisition of multicellularity is a central event in the evolution of Eukaryota. Strikingly, animal Accepted: 30 October 2017 multicellularity coincides with the emergence of three intercellular communication pathways – Notch, Published: xx xx xxxx TGF-β and Wnt – all considered as hallmarks of metazoan development. By investigating Oopsacas minuta and Aphrocallistes vastus, we show here that the emergence of a syncytium and plugged junctions in glass sponges coincides with the loss of essential components of the Wnt signaling (i.e. Wntless, Wnt ligands and Disheveled), whereas core components of the TGF-β and Notch modules appear unaffected. This suggests that Wnt signaling is not essential for cell differentiation, polarity and morphogenesis in glass sponges. Beyond providing a comparative study of key developmental toolkits, we define here the first case of a metazoan phylum that maintained a level of complexity similar to its relatives despite molecular degeneration of Wnt pathways. The emergence of multicellularity was a critical step in the origin of life diversity1. Multicellular lifestyles appeared independently at least 25 times from diverse single-celled ancestors1. However, few lineages accomplished more complex organizations with specialized tissues and/or organs: fungi, land plants, red algae, brown algae and ani- mals2–4. It is commonly accepted that the animal (metazoan) multicellular ancestor likely resulted from the failure of daughter cells to separate after division (colonial hypothesis)2. However, little is known about the mechanisms that allowed the stable transition towards multicellularity. Until now, hints were gleaned through comparison of the genomes of living animals with those of their closest unicellular relatives (Fig. 1a), namely Choanoflagellata and Filasterea5–8. In contrast to animals in which multicellularity is obligate, only few species of choanoflag- ellates and Filasterea are able to transiently form colonies and aggregative stages1,7,9–12. Thus, comparisons of the genetic toolkits of these predominantly unicellular lineages to those of animals, have identified numerous genetic innovations that are now considered as hallmarks of animal multicellularity. Among them, the Delta/ Notch pathway (Fig. 2a) is mainly considered as a key player in cell fate decision while the TGF-β/TGFR (Fig. 3a) and the Wnt/Frizzled (Fig. 4a) modules mediate patterning by controlling cell proliferation and cell motility6,13–18. Co-occurrence of these three ligand/receptor interactions suggests that empowering cell cooperation and cell specialization by efficient cell-to-cell communication was certainly a decisive step in the acquisition of stable multicellularity in animals. Among animals (metazoans), glass sponges (Porifera, Hexactinellida) have a very peculiar tissue organiza- tion19, which makes them highly relevant to address the evolution of cell-to-cell communication during morpho- genesis. Like most metazoans, glass sponges begin life through successive cell divisions (Fig. 1b), which give rise to a hollow blastula made of 16 to 32 cells. However, after the fifth division, cleavages become unequal, cytoplas- mic bridges (named plugged junctions or plugs) are formed between blastomeres, and macromeres progressively merge together giving rise to a syncytial larva (Fig. 1c and d)20. Adult glass sponges mainly consist of a multinu- cleated syncytium as well (the trabecular syncytium), which is connected to different cell types (i.e. archeocytes, choanoblasts and collar bodies) via plugged junctions (Fig. 1e–g)19. This unique syncytial feature, acquired more than 545 million years ago in the last common ancestor of glass sponges21, raises exciting questions regarding its consequences on the developmental toolkit of extant species. 1Aix Marseille Université, CNRS, IRD, IMBE UMR 7263, Avignon Université, Institut Méditerranéen de Biodiversité et d’Ecologie marine et continentale, Station Marine d’Endoume, Marseille, France. 2Department of Genetics and Evolution, University of Geneva, Sciences III, 30 Quai Ernest Ansermet, CH-1211, Geneva 4, Switzerland. 3Aix Marseille Université, CNRS, Centrale Marseille, I2M, Equipe Evolution Biologique et Modélisation, Marseille, France. 4Aix Marseille Université, CNRS, Institute of Developmental Biology of Marseille (IBDM), case 907, 13288, Marseille cedex 09, France. Emmanuelle Renard and Carole Borchiellini contributed equally to this work. Correspondence and requests for materials should be addressed to Q.S. (email: [email protected]) or A.L.B. (email: andre. [email protected]) or C.B. (email: [email protected]) SCIENTIFIC REPORTS | 7: 15383 | DOI:10.1038/s41598-017-15557-5 1 www.nature.com/scientificreports/ Figure 1. Peculiar cell organization and development of glass sponges: Oopsacas minuta. (a) Phylogenetic position of glass sponges (Hexactinellida) among poriferans and metazoans. LCA: Last common ancestor. (b.,f. and h.) Scanning electron micrographs. (c.,d.,e. and g.) Transmission electron micrographs of epoxy sections. (b.) 8-cell stage embryo within maternal tissue43. (c. and d.) Details of embryo showing the fusion of macromeres and the establishment of plugged junctions. (e.) Cytoplasmic continuity between cells through plugged junction. (f. and g.) Collar bodies evidencing a clear cell polarity as shown by the apical pole, ap and the basal pole, bp. (h) Larva showing a clear anterior-posterior axis. The anterior pole and posterior pole are indicated: ap and pp respectively. (i) Adult specimen harboring a clear apico-basal axis. The oral pole (apical pole) and aboral pole (basal pole) are indicated op and abp, respectively. Abbreviations: b, blastomere; c, collar; cb, collar bodies; fl, flagellum; li, lipid inclusion; ma, macromere; nu, nucleus; os, osculum (white arrowhead); pj, plugged junction (grey arrowhead); po, pore particles; ts, trabecular syncytium; y, yolk. Scale: (b.), 43 µm; (c.), 3 µm; (d.,e. and g.), 1 µm; (f.), 4.3 µm; (h.), 50 µm; (i.), 0.5 cm. In order to test the universality of the classical cell-to-cell communication modules of animal development (i.e. Notch, TGF-β and Wnt pathways), we analyzed the transcriptomes of two distantly related glass sponges Oopsacas minuta Topsent, 1927 (Hexactinellida, Lyssacinosida) and Aphrocallistes vastus Schulze, 1886 (Hexactinellida, Hexactinosida) and compared them to those of other sponge lineages (i.e. Calcarea, Demospongiae and Homoscleromorpha) and of other metazoans (Fig. 1a). SCIENTIFIC REPORTS | 7: 15383 | DOI:10.1038/s41598-017-15557-5 2 www.nature.com/scientificreports/ Figure 2. Conservation of the Notch pathway in glass sponges. (a.) Schematic representation of the Notch pathway. In blue the components retrieved in glass sponges, in green the component retrieved in glass sponges but not described in other poriferan lineages until now and in black the element absent in glass sponges and other poriferan. The three successive cleavages of Notch are indicated: S1, S2 and S3. (b.) Domain composition of Notch and Delta proteins. (c.) Alignment of the Delta/Jag cysteine motif. (d.) Domain composition of Furin and ADAM proteins. (e.) Phylogenetic analysis revealing two ADAM10/17 in glass sponges. Black and white triangles represent clades of proteins with a node support higher than 95% and lower than 70%, respectively. (f.) Conservation of the proximal domain of ADAM as shown by the conservation of the cysteine motif. (g.) Domain composition of SuH and CoR1. Results Conserved Notch pathway. In contrast to the previous analysis of A. vastus transcriptome22, we identified the Notch ligand Delta in glass sponges. Indeed, consistent with the analysis of Oscarella carmela, a unique Delta protein was retrieved in glass sponge, whereas five were previously described inAmphimedon genome23. This suggests that a single ligand was SCIENTIFIC REPORTS | 7: 15383 | DOI:10.1038/s41598-017-15557-5 3 www.nature.com/scientificreports/ Figure 3. Conservation of the TGF-β pathway in glass sponges. (a.) Schematic representation of the TGF-β pathway. In blue the components retrieved in glass sponges, in black the element absent in glass sponges and other poriferan and in red the component retrieved in other sponges but not in glass sponges. (b.) Phylogenetic analysis revealing three BMP-like, one TGFβ-like and one unclassified TGF ligand in glass sponges. (c.) Alignment of TGFβ-like motif. In blue the RXXR cleavage site. (d.) Phylogenetic analysis revealing three type I and two type II TGFβ receptors in glass sponges. (e.) Phylogenetic analysis revealing Smad1/5, Smad2/3 and Smad4 in glass sponges. (b.,d. and e.) Black, grey and white triangles represent clades of proteins with a node support higher than 95%, comprise between 70% and 95% or lower than 70%, respectively. (f.) Domain composition of TGFβ ligands and receptors. certainly present in the last common ancestor of sponges and that other paralogs arose later by lineage specific duplications. Computational domain analyses allowed us to confirm the conservation of the overall structure of glass sponge Delta proteins (Fig. 2b). Indeed, the DSL domain, several EGF domains and the transmembrane region were predicted by Interproscan. SCIENTIFIC REPORTS
Details
-
File Typepdf
-
Upload Time-
-
Content LanguagesEnglish
-
Upload UserAnonymous/Not logged-in
-
File Pages11 Page
-
File Size-