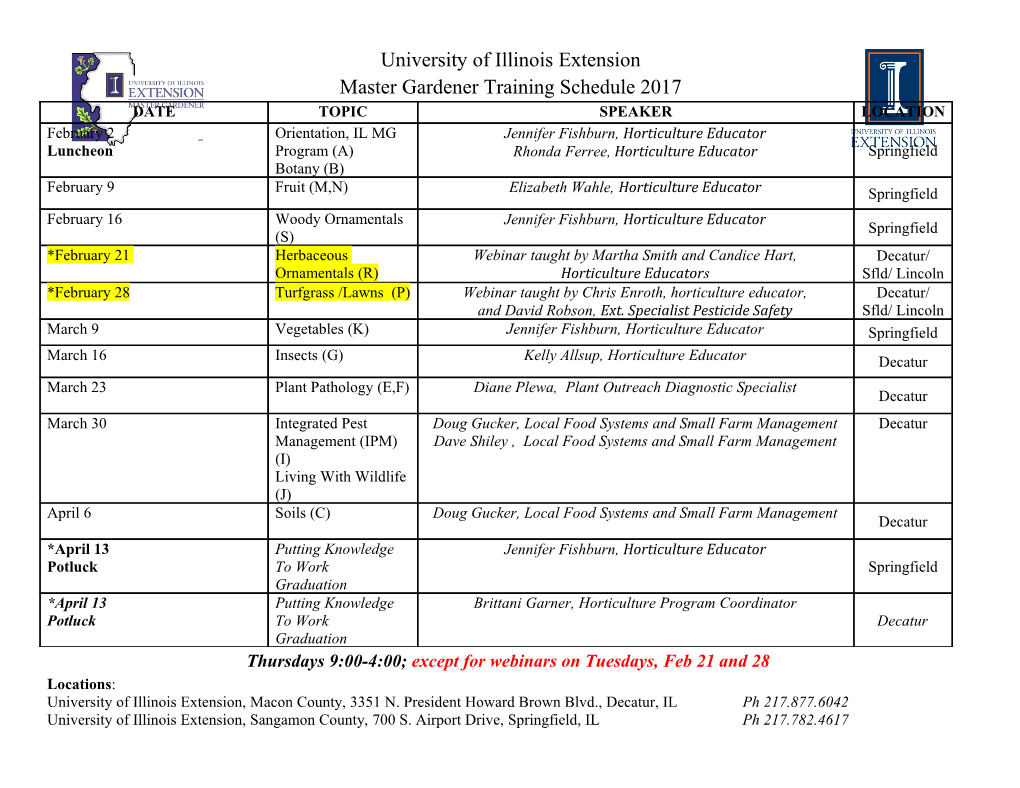
The persistence of waterfalls in fractured rock Michael P. Lamb† and William E. Dietrich Department of Earth and Planetary Science, University of California, Berkeley, California 94720, USA ABSTRACT INTRODUCTION propagation has also been suggested for retreat- ing waterfalls in Australia (Seidl et al., 1996; Although the upstream translation of The upstream propagation of steepened Weissel and Seidl, 1997) and Kaua‘i, Hawai‘i waterfalls is often thought to occur by reaches in bedrock rivers (i.e., knickpoints) is (Seidl et al., 1994, 1997). On Kohala, Hawai‘i, undercutting of resistant strata, collapse, one of the fundamental drivers of landscape evo- others have suggested that headwalls persist and and headwall retreat (e.g., Niagara Falls), lution in hilly and mountainous terrain. Where retreat upstream in the absence of undercutting many propagating waterfalls maintain a knickpoints are near vertical, waterfalls form due to continual generation of stepped water- vertical face in the absence of undercutting. and in some cases can maintain their form while falls and downward abrasion by gravel trans- To explain this observation, we propose that propagating upstream. Why do some waterfalls port (Howard et al., 1994; Lamb et al., 2007). bedrock-fracture geometry exerts a funda- persist rather than decline in height or rotate as Although seepage erosion has been offered as mental control on knickpoint morphology they propagate upstream? To answer this ques- an alternative to plunge-pool erosion (Kochel et and evolution such that vertical waterfalls tion, some researchers have adapted fl uvial-ero- al., 1985; Laity and Malin, 1985; Baker, 1990; can persist during retreat due to toppling sion models based on bed stress, stream power, Pederson, 2001), evidence in support of seepage in bedrock with near horizontal and verti- or saltating sediment to explore the development erosion in bedrock is often ambiguous (Crad- cal sets of joints (e.g., columnar basalt). At and translation of knickpoints (Howard et al., dock and Howard, 2002; Lamb et al., 2006), and a waterfall, rock columns are affected by 1994; Seidl et al., 1994; Whipple and Tucker, seepage fl ow is often incompetent to excavate shear and drag from the overfl owing water, 2002; Bishop et al., 2005; Chatanantavet and collapsed debris away from the headwall (Lamb buoyancy from the plunge pool at the foot of Parker, 2005; Wobus et al., 2006; Berlin and et al., 2006, 2007, 2008). the waterfall, and gravity. We used a torque Anderson, 2007; Crosby et al., 2007; Gasparini Whereas most landscape-evolution models balance to determine the stability of a rock et al., 2007). Although these approaches can treat bedrock as homogeneous (e.g., Howard column and any individual blocks that com- produce an upstream-propagating wave in the et al., 1994; Whipple and Tucker, 2002; Berlin prise the column. Results indicate that rota- landscape, they do not explicitly model the ero- and Anderson, 2007), the mechanics of waterfall tional failure should occur about the base sion processes that occur at a waterfall. More erosion appear to be strongly controlled by bed- of a headwall (and therefore preserve its process-based models involve undercutting or rock strength, jointing, and stratigraphy (Young, form during upstream propagation) where undermining of the waterfall headwall from 1985; Miller, 1991; Weissel and Seidl, 1997; columns are tilted in the downstream direc- either plunge-pool erosion (Stein and Julien, Stein and LaTray, 2002; Frankel et al., 2007). tion or slightly tilted in the upstream direc- 1993; Bennett, 1999; Alonso et al., 2002; Stein Riverbed erosion by abrasion or plucking in tion, depending on the plunge-pool depth. and LaTray, 2002; Flores-Cervantes et al., 2006) general depends on rock strength and joint ori- Flume experiments were performed to test or seepage erosion (Howard and McLane, 1988; entations and spacings (Annandale, 1995; Han- the model, and the model provides a good Dunne, 1990; Lobkovsky et al., 2007; Luo and cock et al., 1998; Whipple et al., 2000; Coleman prediction of the fl ow necessary to induce Howard, 2008). The most well-known example et al., 2003; Wohl, 2008). In bedrock with per- toppling and the morphology of the head- is probably Niagara Falls, where a more resistant vasive vertical joints, it has been suggested that wall. Waterfall-induced toppling explains limestone cap rock appears to be progressively waterfalls might retreat due to toppling of rock the morphology of canyon headwalls in the undercut by plunge-pool erosion, leading to col- columns in the absence of undercutting (Young, volcanic terrain of the northwestern United lapse and upstream propagation of the waterfall 1985; Seidl et al., 1996; Weissel and Seidl, States, where catastrophic paleofl oods (e.g., (Gilbert, 1907). 1997). Such waterfalls may be associated with Bonneville Flood) have carved steep amphi- Although undermining models can explain the particular geologic settings where bedding or theater-headed canyons in columnar basalt. persistence of some waterfalls, many waterfalls foliation planes have been tilted to near vertical This model may also explain similar land- exist that are not undercut (Von Engeln, 1940; (Weissel and Seidl, 1997; Frankel et al., 2007), or forms elsewhere on Earth and Mars, and Young, 1985). Many waterfalls also lack the in volcanic terrains where fl ood basalts develop it can be used to predict the minimum fl ow prominent strong-over-weak stratigraphy that cooling joints (i.e., columnar basalt) (Young, discharge needed to create these features. allows a vertical headwall to persist in weakly 1985; O’Connor, 1993; Lamb et al., 2008). For cohesive sediment (Holland and Pickup, 1976; example, Weissel and Seidl (1997) showed clear Keywords: knickpoint, amphitheater, plucking, Gardner, 1983). The undermining model has evidence of toppled columns at the headwall of toppling, bedrock erosion, Mars. even been questioned for Niagara Falls, where Apsley River Gorge, Australia, where subverti- progressive breakdown of the exposed bedrock cal joints in metasedimentary rocks promote †Current address: Division of Geological and Planetary Sciences, California Institute of Technol- face might be limiting headwall retreat instead of rotational failure. Near-vertical fracturing also ogy, Pasadena, California 91125, USA plunge-pool erosion (Philbrick, 1970, 1974). A might be expected around bedrock canyons E-mail: [email protected] rock-breakdown control on the rate of waterfall in noncompressive tectonic settings due to GSA Bulletin; July/August 2009; v. 121; no. 7/8; p. 1123–1134; doi: 10.1130/B26482.1; 9 fi gures; 1 table. For permission to copy, contact [email protected] 1123 © 2009 Geological Society of America Lamb and Dietrich 114°45'0"W 114°30'0"W B C A Idaho Wood River Malad Malad Gorge Gorge 42°50'0"N Box 42°50'0"N Canyon Blue Lakes Canyon D Woody’s Cove Snake River 42°40'0"N 42°40'0"N m km 01000 0510 114°45'0"W 114°30'0"W CB Malad Gorge CD Woody’s Cove 0 500 m E Blue Lakes Canyon F G Box Canyon G Box Canyon Blind Canyon 0500 m Figure 1. (A) Shaded relief map of the central part of the Snake River Plain, Idaho, showing the locations of three canyons with steep head- walls: Malad Gorge, Box Canyon, and Blue Lakes Canyon. The 30-m-resolution topographic data are from the U.S. Geological Survey. The black lines are 100 m contours. The inset map shows the study location in central Idaho, USA. (B) Shaded relief map of Malad Gorge. C and D labels show the location of the canyon heads in the following photographs. The 10-m-resolution topographic data and 1-m-res- olution orthophotograph are from the U.S. Geological Survey. The black lines are 10 m contours. (C) Photograph of one of the heads of Malad Gorge. The headwall relief is ~50 m. (D) Photograph of the head of Woody’s Cove. A person for scale is circled in the upper right. (E) Shaded relief map of Blue Lakes Canyon. The 10-m-resolution topographic data and 1-m-resolution orthophotograph are from the U.S. Geological Survey. The black lines are 10 m contours. (F) Shaded relief map of Box and Blind Canyons. G label shows the location of the canyon head in the following photograph. The 1-m-resolution airborne laser-swath mapping data were collected by the National Center for Airborne Laser Mapping, and the 1-m-resolution orthophotograph is from the U.S. Geological Survey. (G) Photograph of the headwall of Box Canyon. The headwall relief is ~35 m. All topographic data are projected onto UTM 11N with NAD83 datum. 1124 Geological Society of America Bulletin, July/August 2009 Persistence of waterfalls in fractured rock τ topographically induced stresses (Miller and o Dunne, 1996). A signifi cant motivation for our Fd study is to better understand how, in volcanic terrains, large paleofl oods have rapidly carved P3 η canyons with near-vertical, amphitheater-shaped L headwalls without signifi cant undercutting (e.g., Lamb et al., 2006, 2008). Two prominent exam- P2 ples are Dry Falls from the Missoula Floods of H Washington State, USA (Bretz, 1923; Baker, P 1973) and Asbyrgì Canyon, Iceland (Tómasson, 1 1973). Moreover, large canyons with steep head- walls are abundant on the surface of Mars (e.g., Figure 2. Schematic cartoon showing Fb Baker, 1982), and these might also have been cut fractured basalt and three potential into columnar basalt by large fl oods. points for rotational failure. Two In this paper, we explore the hypothesis that perpendicular joint sets are shown waterfalls can persist in the absence of under- in the schematic, and the third is Hp cutting where river fl ows generate drag forces parallel to the page. Fg P suffi cient to induce toppling in fractured rock θ and transport collapsed debris away from the headwall.
Details
-
File Typepdf
-
Upload Time-
-
Content LanguagesEnglish
-
Upload UserAnonymous/Not logged-in
-
File Pages12 Page
-
File Size-