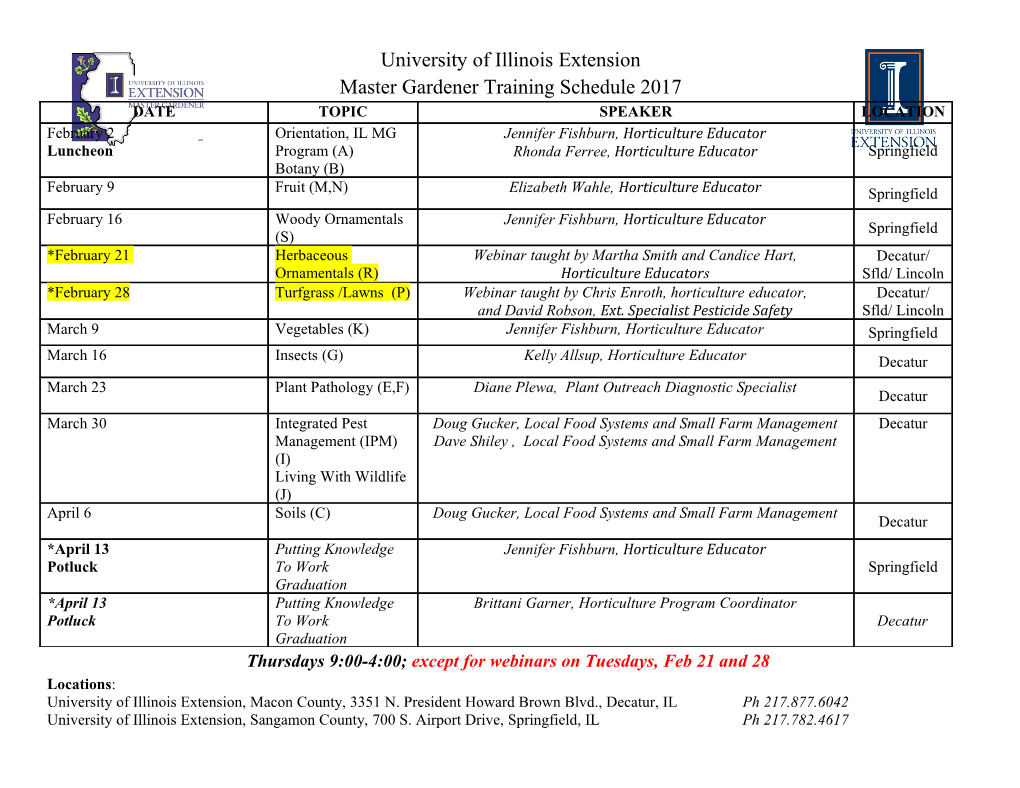
Diana C. G. A. Pinto, João M. P. Pereira, Artur M. S. Silva*1 1.1 Functional Groups of Biomolecules and their Reactions Abstract: This chapter starts with a general introduction on some concepts needed to understand the reactivity of organic functional groups. Elementary reaction mecha- nisms are then presented according to their functionality with relevant biological examples. These reactions explain the vast majority of transformations involving bio- molecules. Finally, two examples on the application of the presented concepts are given, namely the metabolism of fatty acids and reactivity of penicillin. Both of these examples call for various types of reactions showing the diversity and simplicity of biological transformations when analysed step by step. 1.1.1 Functional Groups in Biological Systems The main definition of a functional group in organic chemistry books is as a chemically reactive group of atoms within a molecule that contribute to its characteristic reactivity. Functionality is usually regarded as “implying the presence of heteroatoms and/or unsaturation, but it would not be helpful to attempt to define precisely the limits of application of the term” (IUPAC, Commission on Nomenclature of Organic Chemistry, 1993). Functional group reactivity may be changed by the presence of other neighbouring functional groups but usually behaves uniformly in every molecule where it can be found. There are several common functional groups that are related to families of organic compounds according to their structural features. However, from those functional groups only a few are found in biological systems (Table 1.1.1). The types of bonding found in these functional groups may be explained by the existence of various hybrid atomic orbitals of the carbon atom created from combination of the one 2s and the three 2p orbitals (Table 1.1.2). Diana C. G. A. Pinto, João M. P. Pereira, Artur M. S. Silva: Departamento de Química da Universidade de Aveiro, 3810-193 Aveiro, Portugal, *E-mail: [email protected] Functional Groups in Biological Systems 3 Table 1.1.1: Common functional groups present in biomolecules. In parentheses are the names of the families of compounds, where the group has the highest priority in the compound. C C C=C double bond (alkenes) aromatic ring (arenes) C C C C O N OH R-oxyalkyl (ethers) hydroxyl (alcohols) amino (amines) C S C C C S C SH S sulfhydryl (thiols/mercaptanes) sulfide (thioethers/sulfides) disulfide (disulfides) O O N C C C C C H C C formyl (aldehydes) dialkylcarbonyl (ketones) imino (imines) O O O C C C C C OH C O C N carboxyl (carboxylic acids) R-oxycarbonyl (ester) aminocarbonyl/carboxamide (amides) O O O O C C C P C P O C S C O O O O sulfanyl O carbonyl (thioesters) alkyl phosphate (phosphates) acyl phosphate (mixed anhydrides) 4 Functional Groups of Biomolecules and their Reactions Table 1.1.2: Possible orbital combinations in the carbon atom, their spatial configuration and geometry with inter-bond angles. Combination Hybrid result Geometry 1 x 2s + 3 x 2p 4 x sp3 tetrahedral 1 x 2s + 2 x 2p 3 x sp2 trigonal planar 1 x 2s + 2 x 2p 2 x sp linear All of the hybrid orbitals may be used to form σ (sigma) molecular orbitals by fusion with s or with other hybrid orbitals from other atoms (according to Molecular Orbital Theory). If there are remaining 2p orbitals in the carbon atoms (in the case of sp2 and sp hybrid orbitals) they are used to form π (pi) molecular orbitals by lateral combination with other adjacent 2p orbitals (Fig. 1.1.1). A simple bond is formed with a single σ-bond; the double bond is formed with a σ-bond and a π-bond; the triple bond is formed with a σ-bond and two π-bonds. Figure 1.1.1: A simplified representation of the spatial distribution of two adjacent p orbitals (a) and their combination into a π bond (b) between two sp2 carbon atoms. The π-bonds in a triple bond would be along orthogonal planes to each other. Acids and Bases Versus Electrophiles and Nucleophiles 5 These hybridisations have several consequences, such as the electron density of a π-bond lying above and below the plane of the bonding atoms (Fig. 1.1.1), resulting in greater exposure for a reaction. Simultaneously, with the increasing s character of the hybrid orbital: – the formed bond length decreases; – the polarity of a C-H bond increases; – breaking a bond between carbon and a more electronegative atom is more dif- ficult (e.g. the C-O bond in isopropanol is easier to cleave than the C-O bond in isopropenol). The electronegativity of an element can also be an important factor to explain some functional groups reactivity. For instance, alcohols, ethers, amines, thiols, sulfides, disulfides and phosphates (Table 1.1.1) all have a carbon forming a single bond with a more electronegative atom, causing the carbon to bear a partial positive charge (δ+). These modifications affect both the σ- and π-bonds, although in the case of some π-bonds the resonance effect should also be considered. The carbonyl group can be classically treated as a resonance hybrid represented by two resonance structures (Scheme 1.1.1), which contributes to the reactivity of the compounds that have this functional group (aldehydes, ketones, carboxylic acids, esters, thioesters, amides, acyl phosphates). Resonance is possible whenever movement of electrons are allowed within the same molecule without movement of atoms. − O O O δ or + C C C δ Scheme 1.1.1: Carbonyl group resonance structures and equivalent notation, explicitly showing the bond polarity with partial charges. 1.1.2 Acids and Bases Versus Electrophiles and Nucleophiles Acids and bases are very important in biological transformations, as most require some form of acid or basic catalysis to occur. The simplest acid-base theory is the Brønsted-Lowry theory, which states that acids are molecules that donate protons (hydrogen ions, H+) and bases/alkalis are molecules that accept protons. For example, a carboxylic acid can donate a proton to a base, such as an amine, in a reversible proton-transfer reaction (Scheme 1.1.2). 6 Functional Groups of Biomolecules and their Reactions O H O H + N + N H R OH R1 H R O R1 H acid base conjugate base conjugate acid Scheme 1.1.2: Example of a proton transfer reaction. This specific reaction explains why it is difficult for condensations to happen directly between an amine and a carboxylic acid, as the non-ionic forms of these molecules are more reactive (Chapter 1.1.4.5). Acids can differ in their ability to donate protons, being classified as strong or weak according to the extent of deprotonation. A strong acid will have a stable conjugate base (or weak conjugate base), resulting in ready donation of a proton. Table 1.1.3 lists the acidity of some typical functional groups (water and ammonium acidity are also given for comparison). The acidity is measured by the acidity constant, Ka or by its pKa (Scheme 1.1.3), where a stronger acid has a smaller pKa and a weaker acid has a larger pKa. The same approach can be applied to bases and their strength. - + HA + H2O A + H3O Scheme 1.1.3: Acidity constant and pKa. The problem with the Brönsted/Lowry definition is that it only covers the compounds that donate or accept protons. The more general and widely used model is the Lewis definition. A Lewis acid is a molecule that accepts a pair of electrons and a Lewis base is a molecule that donates a pair of electrons. To accept electrons, a Lewis acid must have a vacant low-energy orbital. As a consequence, many species, including H+ itself, metal cations such as Mg2+ and Zn2+, and neutral species such as boron trifluoride (BF3) and carbon dioxide (CO2) are Lewis acids. Lewis acids and bases are involved in many biological reactions, such as the transformation of carbon dioxide into hydrogen carbonate (Scheme 1.1.4). Lewis bases use unshared electrons to form new bonds with other atoms and are usually referred as nucleophiles (“nucleus-loving”, versus Lewis acids as electrophiles, “electron-loving”). The terms “electrophile” and “nucleophile” are commonly used Acids and Bases Versus Electrophiles and Nucleophiles 7 in organic and bioorganic transformations. Electrophiles are either positively charged or neutral and have at least one positively polarised, electron-poor atom. Conversely, nucleophiles are either negatively charged or neutral and have a lone pair of electrons that can be donated. Table 1.1.3: Relative acidity strengths of some functional groups and common reactants. Functional group Example pKa carboxylic acid O 4.76 CH 3COH ammonium + 9.26 NH4 alkylthiol CH 3SH 10.3 alkylammonium ion + 10.66 CH 3NH3 β-keto ester O O 10.6 CH 3CCH2COCH 3 water 15.74 H2O alcohol 16.0 CH 3CH 2OH ketone O 19.3 CH 3CCH3 thioester O 21 CH3CSCH 3 ester O 25 CH3COCH 3 δ− O carbonic O δ+ anhydrase HO + C C HO O O δ− Lewis base Lewis acid Scheme 1.1.4: Example of a biochemical reaction involving a Lewis acid and a Lewis base. Reversible conversion of carbon dioxide to hydrogen carbonate can occur rapidly in the active site of a carbonic anhydrase, the equilibrium being regulated by factors such as pH. 8 Functional Groups of Biomolecules and their Reactions 1.1.3 Stereoisomerism and Chirality Stereoisomers having the spatial orientation of bonds as their unique difference, maintaining the same composition of atoms and bonds.
Details
-
File Typepdf
-
Upload Time-
-
Content LanguagesEnglish
-
Upload UserAnonymous/Not logged-in
-
File Pages42 Page
-
File Size-