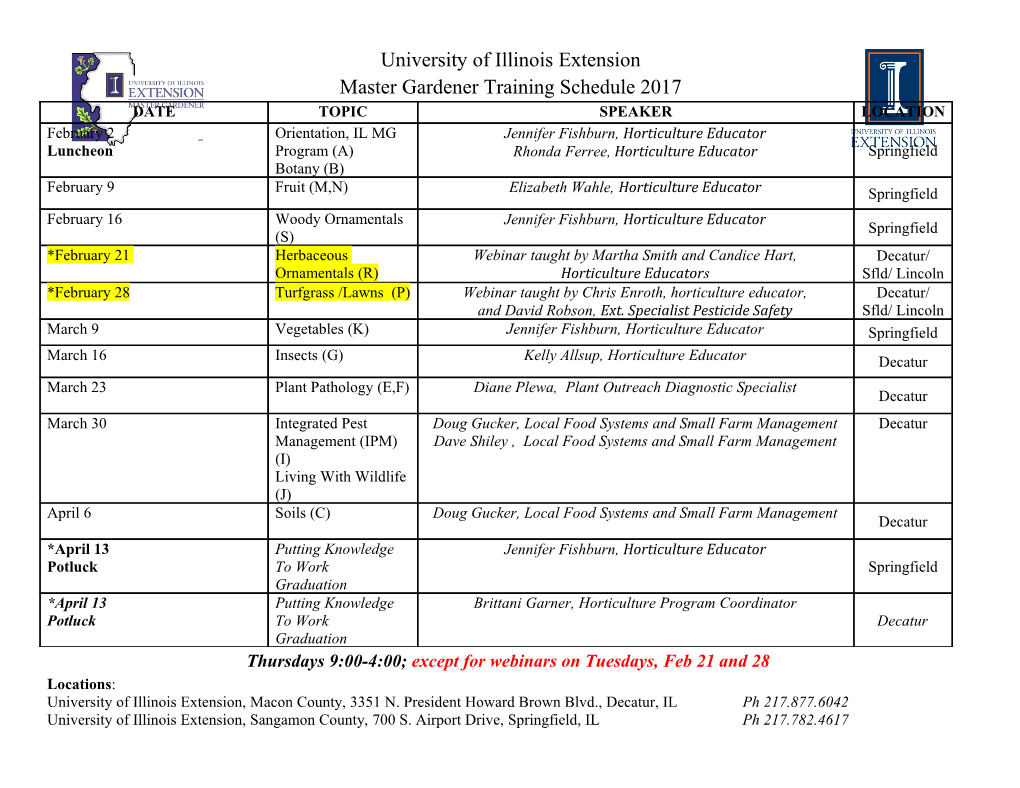
RIT Electrical Engineering Department EE Freshman Practicum Week 8 AM Modulation and the AM Receiver The concept of modulation and radio transmission is introduced. An AM receiver is studied and the constructed on the prototyping board. The operation of the AM receiver is observed on the oscilloscope at various test points in the prototype circuit. Introduction to Radio Transmission and Reception The typical person can hear sounds which range from a value of 20 Hz to about 20 kHz. But an AM radio receiver in your car must operate in the broadcast frequency range from about 530 kHz to 1680 kHz. The function of the AM receiver is to decode an incoming radio carrier signal and to output the original audible signal that we hear. You may wonder why someone would go through all the trouble of changing the transmitted signal if the resulting output (sound) would be the same as the signal before it was broadcasted. There are two main reasons for why this is so. If there were twenty people transmitting signals between 20 Hz and 20 kHz, the probability of one signal interfering with another would be very high. The second reason is that signals at low carrier frequencies would require very large antennas, as the length of the antenna has an inverse relationship with the transmission frequency. So now that we know why we can’t broadcast a signal without altering it in some way, let’s study how to do it. An easy way to do this is through a technique called amplitude modulation (AM). An input signal, referred to as a message signal, mn(t) is simply multiplied by a another signal, called the carrier which is at a much higher frequency. The carrier is usually denoted Accos(ct). The result is called the modulated signal. An example of this is shown in Figure 8.1. Notice that the variation in the amplitude of the envelope of the modulated signal is the message signal! The modulated signal is then sent to a transmitter. Afterwards, it travels through the air until it is intercepted by an antenna on the AM receiver. Message Signal Acmn(t)cos( ct) mn(t) Carrier Signal Accos( ct) Figure 8.1: Example of AM Modulation The AM Receiver An AM receiver (like the one found in a car or in a portable radio) is capable of picking up the radio waves transmitted from a radio station and transforming them into audible signals suitable to the human ear. As shown in Figure 8.2, many stations may be transmitting different signals simultaneously, and therefore the AM receiver must be capable of focusing on the specific station of your choice (in circuit parlance, this is referred to as “tuning”). 63 RIT Electrical Engineering Department EE Freshman Practicum Station 1 Station 2 Station 3 Receiver Figure 8.2: Multiple Stations near a Single Receiver. Let’s look at a block diagram of a conventional AM receiver in Figure 8.3 that might be found in your car. The receiver must be able to tune to a particular frequency while having the ability to reject the rest so that we only listen to one station at a time. The receiver must also convert the modulated signal into the original signal. This conversion is accomplished through a peak detector. After the original signal is recovered it will be necessary to amplify it so that one can hear the signal on a speaker or headset. Modulated Radio Audible Output Signal Tuning Peak Antenna Amplifier Speaker Network Detector Functions: Receives the Tunes to a Detects the Amplifies the Signal sent to a modulated particular original signal signal to an speaker. signal frequency acceptable level Figure 8.3: Functional Diagram of the AM Receiver. Let’s take a closer look at each of the blocks in Figure 8.3. The antenna will sense all radio signals flowing through the air waves. Exactly how the antenna is able to ‘pick up’ radio waves from the air is best explained by an analogy. Imagine a fisherman is sitting on a boat, as in Figure 8.4. If a person near the lake shore drops a stone in the water, ripples travel through the water and eventually reach the fishing string. When they do, the fishing string vibrates. The antenna behaves very much like the fishing string, and electrons inside it vibrate when radio waves come in contact with it. …and cause the fishing string to swing Stone is dropped Ripples travel in lake… towards boat… Figure 8.4: Vibrating Fishing String Analogy. 64 RIT Electrical Engineering Department EE Freshman Practicum The tuning network allows one to select a specific radio station. The tuning network is comprised of an inductor and a capacitor in parallel (Figure 8.5) and is called the LC network. The idea of resonance which was introduced in weeks one and two will be utilized once again. However, this time resonance will not be used to make an oscillating circuit. Instead, it will be used as a band-pass filter. This means that signals at a frequency f will be passed while the rest will be rejected, or “filtered” out as the name suggests. The frequency response of the LC network is also shown in Figure 8.5. The formula for finding this resonant frequency is given by equation (8.1). ) B d ( n i a G f Frequency (Hz) Figure 8.5: Schematic of the LC Network (Left), and its Frequency Response (Right). ω 1 f = = (8.1) 2π 2π LC Where L is defined as the inductance, C is the capacitance and 2 is a scaling factor which converts (with units of radians per second) into f (with units of Hz). With the radio station of interest selected, it comes time to recover the original signal. This step is called de-modulation and is done using a peak detector. The circuit is comprised of a diode, a resistor and a capacitor as shown in Figure 8.6. Recall the fact that a diode is a rectifier, which means that it will only pass a signal in one direction. Therefore, as the input voltage applied to the diode grows, so does the voltage at the output. This output voltage will store charge on the capacitor. When the input voltage drops below the voltage of the capacitor, the diode no longer conducts as it does not allow current to flow in the reverse direction. At this point, the capacitor begins to discharge through the resistor resulting in a voltage drop at the output. This will continue on until the voltage at the input exceeds the voltage across the capacitor. The cycle is repeated continuously. If the capacitor and resistor values are chosen carefully, the output of the peak detector will trace the envelope of the modulated signal very closely as shown in Figure 8.6. The result is the original transmitted signal. 65 RIT Electrical Engineering Department EE Freshman Practicum ) + V ( A m (t)cos( t) e c n c g a t l - o V Time (sec) Figure 8.6: Schematic of the Peak Detector (Left), and its Input and Output (Right). The signal that comes out of the peak detector is typically very small, usually on the order of hundreds of micro-Volts at best. This is not loud enough to be heard by the human ear, so it becomes necessary to amplify this signal so that it can drive a speaker. There are many amplifier configurations which range from something as simple as a couple of transistors to something complex, like an operational amplifier, also known as an op-amp. You will learn more about transistors in the sophomore practicum course. In this exercise, we will use a companion op-amp of the one used in the Wein-Bridge oscillator during weeks one and two. Together, these functional blocks result in the AM receiver of Figure 8.7. The functional blocks are labeled according to Figure 8.3. Please note that since the speaker is already soldered into the PCB for use in the Wein-Bridge oscillator it will not be used in the prototype of the AM receiver. However, “Test Point 3” represents the signal that would be sent to the speaker. Test Point 2 Test Point 1 Test Point 3 Figure 8.7: Schematic of the AM Receiver to be prototyped. Let’s take a closer look at the op-amp. Resistors R9 and R10 are configured in what is called the non-inverting configuration which keeps the gain of the op-amp at a constant value of 4.7. To derive this relationship, the nodal analysis technique which was introduced in week 3 will be used. However, before we can do this a couple of simplifying conditions must be stated. This first assumption is that the gain of the op-amp is infinity. The second assumption is that the output resistance of the op-amp is zero. Next, we assume that the voltage at both inputs is equal. The last assumption states that the input impedance is infinite; this allows us to say that the current flowing into the inputs of the op-amp is zero. 66 RIT Electrical Engineering Department EE Freshman Practicum Keeping these things in mind, one can write the following at pin 2: V2 − 0 V2 −V3 I 2 + I 3 = + = 0 (9.2) R10 R9 Since our third assumption says that the input voltages are equal, this means V2 = V1. With simple re-arrangements we can see that: ≈ ’ V V V R ∆ 1 1 3 3 3 9 V2 ∆ + = Gain = = = 1+ = 4.7 (9.3) « R10 R9 R9 V2 V1 R10 Lab Exercise Build the AM receiver shown in Figure 8.7.
Details
-
File Typepdf
-
Upload Time-
-
Content LanguagesEnglish
-
Upload UserAnonymous/Not logged-in
-
File Pages8 Page
-
File Size-