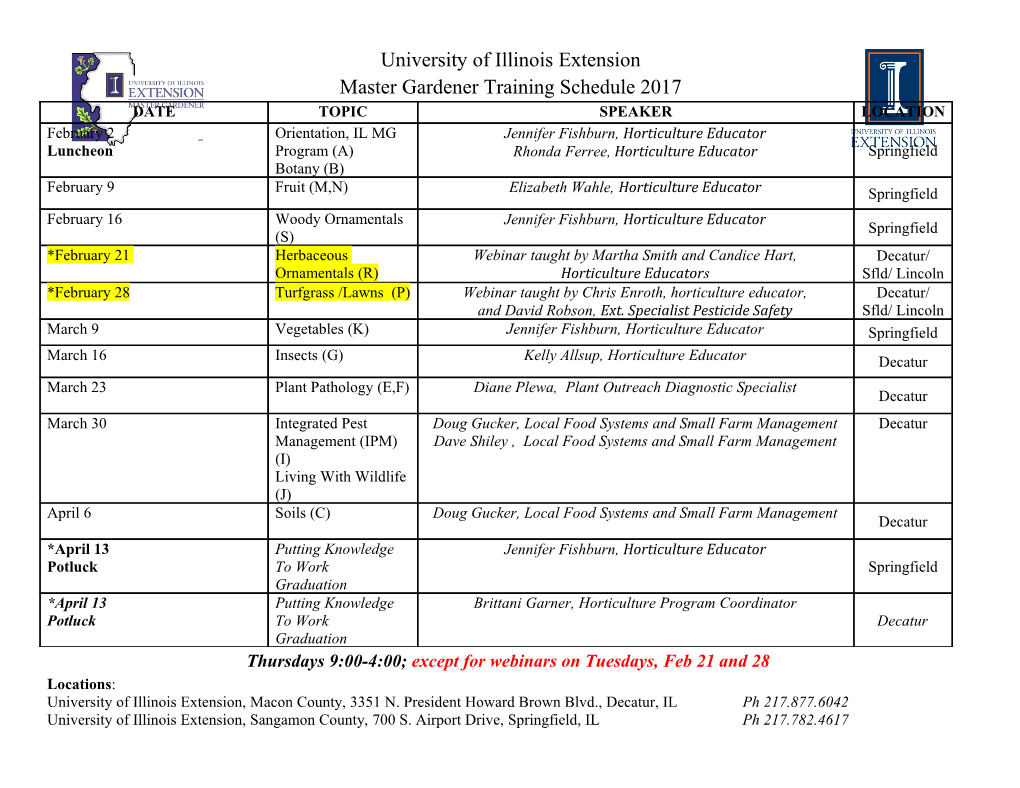
REPORTS 8. C. Resini, T. Montanari, G. Busca, J.-M. Jehng, 17. M. Salmeron, R. Schlögl, Surf. Sci. Rep. 63, 169 (2008). für Synchrotronstrahlung for support of in situ XPS I. E. Wachs, Catal. Today 99, 105 (2005). 18. T. I. T. Okpalugo, P. Papakonstantinou, H. Murphy, measurements; and M. A Smith for helpful discussions. 9. T. G. Alkhazov, E. A. Ismailov, A. Yu, A. I. Kozharov, J. McLaughlin, N. M. D. Brown, Carbon 43, 153 (2005). Supported by the CANAPE project of the 6th Framework Kinet. Katal. 19, 611 (1978). 19. L. M. Madeira, M. F. Portela, Catal. Rev. 44, 247 (2002). Programme of European Commission and the ENERCHEM 10. M. S. Kane, L. C. Kao, R. K. Mariwala, D. F. Hilscher, 20. F. Atamny et al., Mol. Phys. 76, 851 (1992). project of the Max Planck Society. H. C. Foley, Ind. Eng. Chem. Res. 35, 3319 (1996). 21. M. L. Toebes, J. M. P. van Heeswijk, J. H. Bitter, 11. M. F. R. Pereira, J. J. M. Órfão, J. L. Figueiredo, A. J. van Dillen, K. P. de Jong, Carbon 42, 307 (2004). Appl. Catal. A 218, 307 (2001). 22. A. M. Puziy, O. I. Poddubnaya, A. M. Ziatdinov, Appl. Surf. Supporting Online Material www.sciencemag.org/cgi/content/full/322/5898/73/DC1 12. J. Zhang et al., Angew. Chem. Int. Ed. 46, 7319 (2007). Sci. 252, 8036 (2006). Materials and Methods 13. G. Mestl, N. I. Maksimova, N. Keller, V. V. Roddatis, 23. S. J. Clark et al., Z. Kristallogr. 220, 567 (2005). R. Schlögl, Angew. Chem. Int. Ed. 40, 2066 (2001). 24. J. P. Perdew, K. Burke, M. Ernzerhof, Phys. Rev. Lett. 77, Figs. S1 to S6 Table S1 14. See supporting material on Science Online. 3865 (1996). 15. J.-H. Zhou et al., Carbon 9, 1379 (2007). 25. We thank the Max Planck Society; U. Wild, References 16. K. D. Chen, E. Iglesia, A. T. Bell, J. Phys. Chem. B 105, A. Klein-Hoffmann, and J. Thielemann for technical 17 June 2008; accepted 21 August 2008 646 (2001). assistance; Berliner Elektronenspeicherring-Gesellschaft 10.1126/science.1161916 laboratory introduced the concept of organo– Merging Photoredox Catalysis with singly occupied molecular orbital (SOMO) ca- talysis, a one-electron mode of activation that has enabled the development of several useful trans- Organocatalysis: The Direct formations (6–10). Given the widespread success of both elec- Asymmetric Alkylation of Aldehydes tron transfer catalysis and organocatalysis, we recently questioned whether it might be possible David A. Nicewicz and David W. C. MacMillan* to merge these two powerful areas, with the goal of solving long-standing, yet elusive problems in Photoredox catalysis and organocatalysis represent two powerful fields of molecule activation that chemical synthesis. More specifically, as a blue- have found widespread application in the areas of inorganic and organic chemistry, respectively. print for reaction invention, we hoped to exploit We merged these two catalysis fields to solve problems in asymmetric chemical synthesis. the lessons of photoredox enzymatic catalysis Specifically, the enantioselective intermolecular a-alkylation of aldehydes has been accomplished (11), wherein a series of consecutive low-barrier, using an interwoven activation pathway that combines both the photoredox catalyst Ru(bpy)3Cl2 open-shell steps are energetically preferred to high- (where bpy is 2,2′-bipyridine) and an imidazolidinone organocatalyst. This broadly applicable, yet barrier, two-electron pathways. On this basis, we previously elusive, alkylation reaction is now highly enantioselective and operationally trivial. hypothesized that the enantioselective catalytic a-alkylation of aldehydes (12–15), a widely sought ature’s ability to convert solar energy to orbital of an electron-rich substrate reacts with yet elusive transformation, might be brought to chemical energy in photosynthesis has the lowest unoccupied molecular orbital of an fruition via the marriage of inorganic electron Ninspired the development of a host of electron-deficient partner. Recently, however, our transfer and organic catalysis (Fig. 1). photoredox systems in efforts to mimic this process. Arguably the most studied one-electron 2+ photoredox catalyst has been Ru(bpy)3 (where bpy is 2,2′-bipyridine): an inorganic complex that has facilitated important advances in the areas of energy storage, hydrogen and oxygen evolution from water, and methane production from carbon dioxide (1, 2). Given its proven ability to mediate electron transfer, it is surpris- 2+ ing that Ru(bpy)3 has not found a substantial application in organic synthesis, wherein a large number of fundamental reactions rely on the gen- eration and exploitation of radicals or single- electron intermediates (3). Over the past decade, the field of organo- catalysis has grown at a dramatic pace, provid- ing more than 130 chemical reactions that rapidly facilitate enantioselective C–C, C–O, C–N, and C–halogen bond formation (4, 5). Whereas a broad range of reaction types have recently suc- cumbed to this mode of catalysis (including aldol, Friedel-Crafts, and cycloadditions), it is important to consider that nearly all organocatalytic bond constructions are restricted to two-electron path- ways, wherein the highest occupied molecular Contribution from Merck Center for Catalysis, Department of Chemistry, Princeton University, Princeton, NJ 08544, USA. Fig. 1. Merging amine catalysis and organometallic photoredox catalysis to enable asymmetric *To whom correspondence should be addressed. E-mail: organic transformations. Me, methyl; R, generic organic substituent; FG, electron-withdrawing func- [email protected] tional group. www.sciencemag.org SCIENCE VOL 322 3 OCTOBER 2008 77 REPORTS We proposed that two interwoven catalytic From the outset, we understood that the utility This new asymmetric alkylation protocol was cycles might be engineered to simultaneously of this alkylation reaction would rely on the iden- first examined using octanal and bromo dieth- generate an electron-rich enamine from the con- tification of an amine catalyst that could gener- ylmalonate as the coupling partners, along with densation of an aldehyde and an amine catalyst ically enforce high levels of enantiocontrol in the a catalyst combination of Ru(bpy)3Cl2 (1) and and an electron-deficient alkyl radical via reduc- coupling of the pivotal p-rich enamine with a imidazolidinone 6, and a 15-W fluorescent light tion of an alkyl bromide with a Ru photoredox diverse array of electron-deficient radicals. On source (Table 1) (27). To our great delight, pre- catalyst (Fig. 2). Given that electron-deficient rad- the basis of density functional theory (DFT) cal- liminary studies revealed the successful execu- icals are known to rapidly combine with p-rich culations (25, 26), we proposed that the imid- tion of our dual-cycle design ideals to provide olefins to forge even the most elusive C–C bonds azolidinone catalyst 6 should selectively form an (R)-2-malonyloctanal with excellent levels of (16, 17), we hoped that this dual-catalysis mech- enamine 8 (DFT-8), that projects the 2p electron enantiocontrol and reaction efficiency [entry 1, anism would successfully converge to enable system away from the bulky tert-butyl group, 93% yield, 90% enantiomeric excess (ee)]. Ex- the direct coupling of aldehydes with a-bromo whereas the electron-rich olefin will selectively periments that probe the scope of the aldehyde ketones or esters. As a critical design element, populate an (E)-configuration to minimize non- component in this new alkylation reaction are we presumed that the use of a suitable chiral bonding interactions with the imidazolidinone ring summarized in Table 1 (entries 1 to 6). Chemical amine catalyst would induce high enantiose- (Fig. 2). In terms of enantiofacial discrimination, functionalities that are often prone to either lectivity. Moreover, we recognized that the in- the calculated DFT-8 structure also reveals that the oxidation or reduction (e.g., olefins, esters, car- teraction of a SOMOphilic enamine with an methyl group on the catalyst system will effec- bamates, and arenes) were found to be inert to electron-deficient radical is the converse mecha- tively shield the Re face of the enamine, leaving these mild redox conditions (entries 2 to 5, 66 to nism to our previously described SOMO activa- the Si face exposed for enantioselective radical 92% yield, 90 to 95% ee). Moreover, the steric tion studies. As such, a complementary array of addition. We have found that the trans methyl, demand of the a-formyl substituent has little catalytic bond constructions should be possible. tert-butyl 2,5-disubstituted imidazolidinone 6 is impact on the efficiency and enantioinduction A detailed description of our dual-catalysis an excellent enamine catalyst for transforma- of the alkylation process (entries 1 and 4, sub- aldehyde alkylation is presented in Fig. 2. It tions performed at room temperature. Specifically, stituent is n-hexyl versus cyclohexyl, 83 to 93% 2+ has long been established that Ru(bpy)3 (1) catalyst 6 provides excellent levels of kinetic yield, 90 to 95% ee), a point that is underscored will readily accept a photon from a variety of enantiocontrol yet does not readily participate by the successful use of adamantyl acetaldehyde 2+ light sources to populate the *Ru(bpy)3 (2) in enamine formation with the 2,2′-disubstituted (entry 6, 63% yield, 93% ee). metal-to-ligand charge transfer (MLCT) excited aldehyde-alkylation adduct, a step that would A broad array of electron-deficient a-bromo 2+ state (1, 2). Although *Ru(bpy)3 (2) can func- erode product enantiopurity via epimerization. carbonyls
Details
-
File Typepdf
-
Upload Time-
-
Content LanguagesEnglish
-
Upload UserAnonymous/Not logged-in
-
File Pages4 Page
-
File Size-